As biosensor technology continues to advance, it is being applied in a variety of new and exciting research areas. Miniaturization of sensors, wireless data transmission, and advances in automated signal processing have made it easier and more cost effective than ever to acquire multimodal physiological data and use it to understand human behavior.
A similar level of excitement existed during the early days of computer programming as computers became more powerful and less expensive. However, it was at this same time that the proverb “garbage in, garbage out” was coined to describe that flawed data inputted into a computer program would result in equally nonsensical output data [1].
This general principle has similar implications within human behavior research. No matter the advances in biosensor technology, conclusions drawn on shaky empirical premises will not hold up to scientific scrutiny. Therefore, the opportunity for educators to promote critical thinking skills, and the values embodied by scientific inquiry, can help shape a future generation of human behavior researchers grounded in rigorous research practices. In other words – excellence in, excellence out.
Below we will discuss 5 teaching tips that can help you foster a positive and optimal learning environment for students, and maximize the impact of your lessons.
1. Connect theoretical concepts to practical applications
Lifting theoretical concepts from the pages of a textbook and setting them into action can help engage students and promote memory retention [2]. As an educator, one approach to making more of these connections is to think about non-obvious, practical, applications – both big and small.
Take the example of the physiological phenomenon whereby exaggerated breathing is associated with momentary changes in arousal that can be measured using an electrodermal activity (EDA) sensor.
Theoretical concept: voluntarily inhalation and subsequent breath-holding in humans results in a momentary increase in arousal (stress) which is associated with a rise in skin conductance level (SCL) [3].
Practical application: these phenomena can be implemented as a verification step when a research participant is fitted with sensors before data collection begins. In this way, a researcher can piggyback on an innate bodily reaction in order to ensure the electrodes of the EDA sensor are picking up meaningful physiological information.
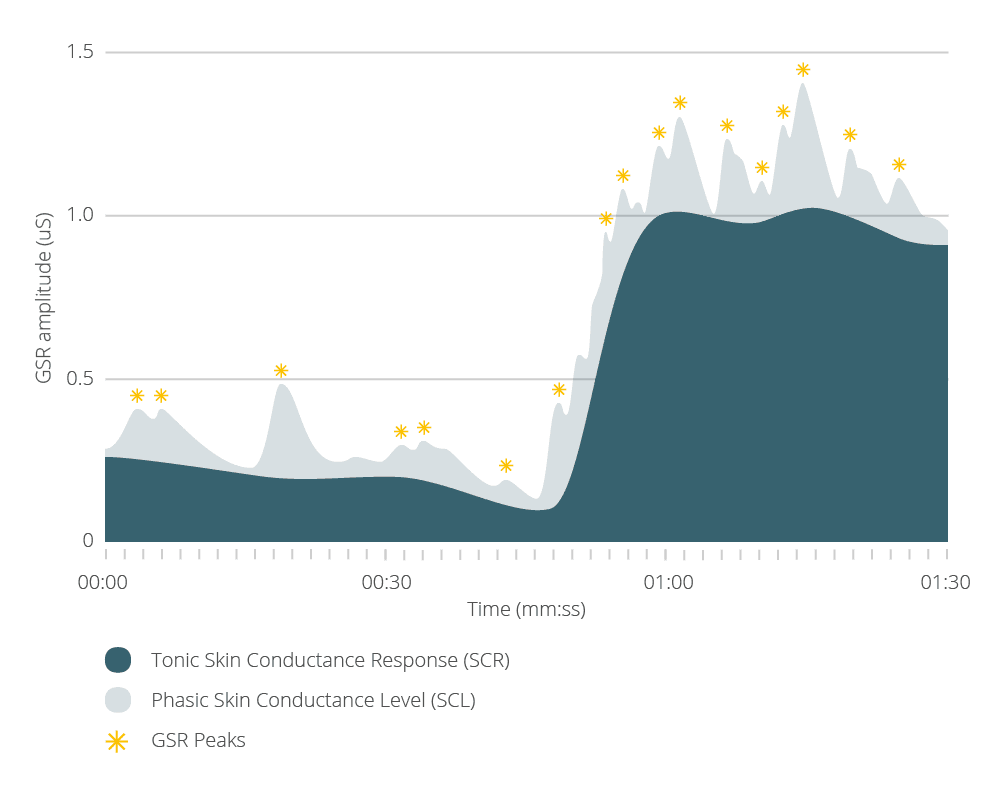
This example underlines the potential for a small concept to be used by students in practical ways to develop a broader understanding of physiology. Bringing information to life makes it more enjoyable to learn, relatable to the real world, and more easily understood.
2. Provide examples with real-world data visualizations
Nearly all human behavior research concepts can be described in words, yet many remain elusive unless visualized in some way. Consider human perceptual biases which are notoriously challenging to observe and explain because they remain largely hidden from conscious processing or subjective experience.
Here is a text-based explanation of the main visual attention biases [4, 5, 6]:
- Directed attention is the tendency to meet the gaze of a person looking at an object within a scene.
- Social cues bias is the tendency to fixate on objects conveying social information within a scene.
Now let’s visualize each of these as they manifest in the eye tracking data from a research study that presented static images to 90 participants.
Social cues bias visualized using a static bee-swarm heatmap of all fixations made across all study participants:
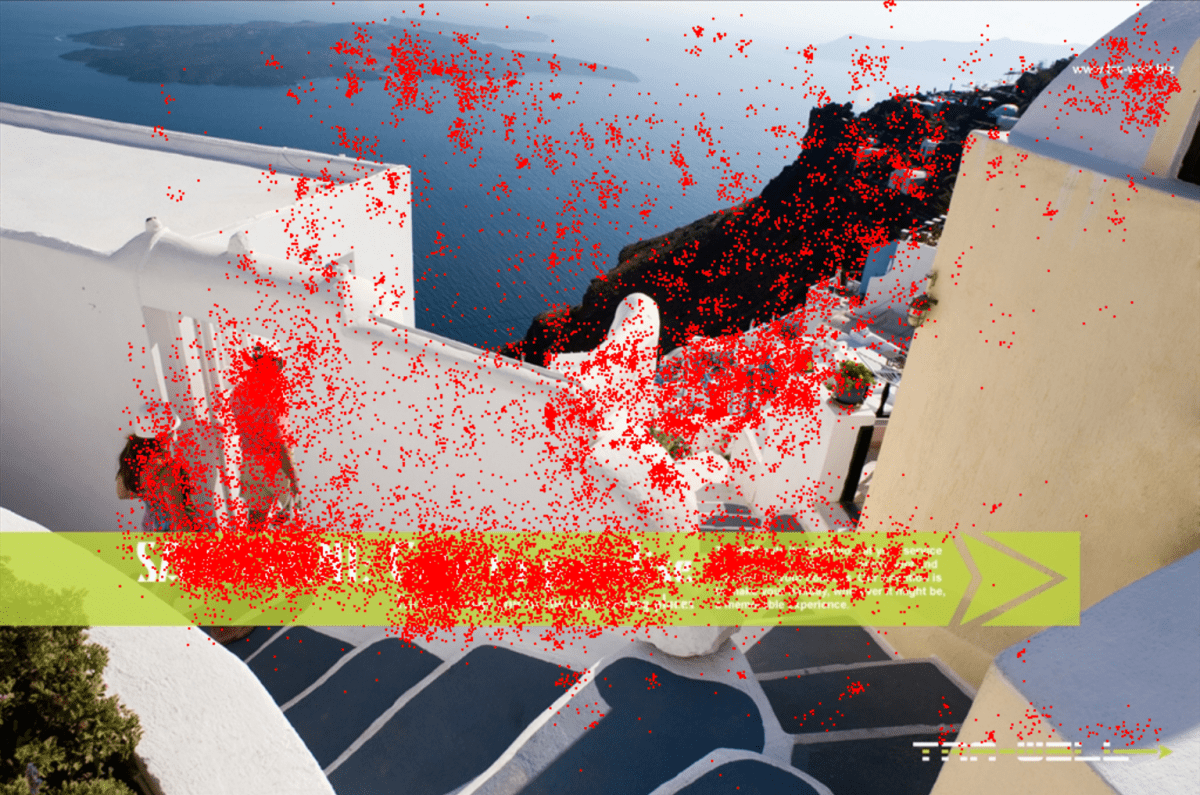
Promoting a thorough understanding of these biases is important due to their universal nature and ability, and if not accounted for, to unintentionally influence experimental findings. Merely describing them though is nowhere near as explanatory as witnessing them in action using data visualizations. Seeing helps in not only believing, but also understanding.
3. Use Socratic questioning to foster critical thinking
At its core, student learning within experimental psychology is based on the need to explain phenomena at the intersection of physiology and psychology. In an inquiry-centered classroom, educators provide as many opportunities as possible for students to construct their own explanations and arguments, then evaluate those explanations and arguments based on evidence.
One powerful technique to facilitate this process is the deliberate application of Socratic questioning during open-ended learning experiences. First championed by the ancient Greek philosopher Socrates, this approach enables students to discover answers themselves rather than the answers being dictated by a teacher.
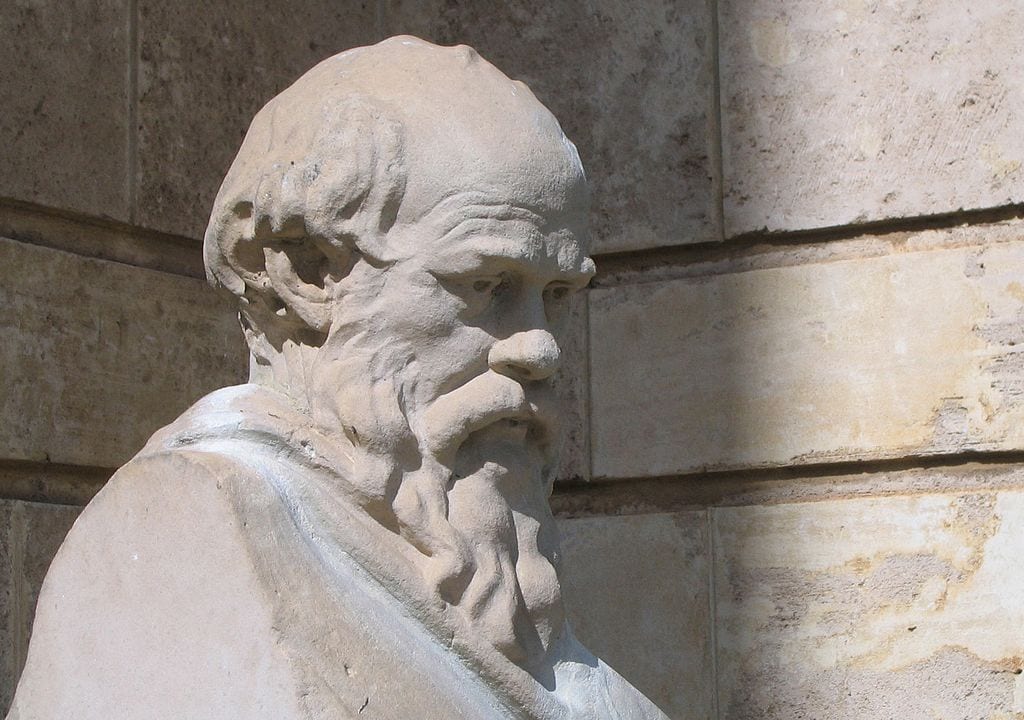
Within biosensor research, strategies such as probing, prompting, and re-directioning can aid in solidifying a fundamental understanding of challenging concepts.
Consider a fundamental aspect of many research studies: biosensor data that describes the behavior of a small group of individuals is used to make inferences about population-wide behavior.
One of the key factors that either enables or limits the validity of this inferential reasoning is the quantity and representativeness of the individuals within the study sample. Students can be probed in many ways to reveal underlying assumptions about how their own emotional experience maps onto that of other individuals or the population at large.
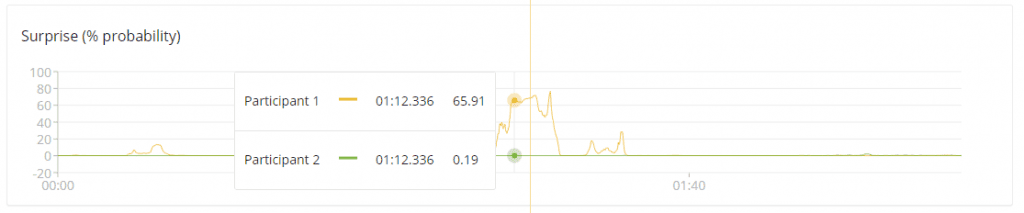
Without prior experience collecting and analyzing data, it is often easy to assume that the data from a single participant is indicative of the entire study sample or that the entire study sample is representative of an entire population. Socratic questioning enables students to distinguish what they know and understand from what they don’t know or understand.
4. Tie it back to the scientific research process
When learning about complex concepts, it can be difficult for students to place the parts within a bigger picture, and understand how each works together. As a result, it’s important to present details within a relateable framework, so that the full meaning can be understood.
The following example refers to this in the context of biosensor-based research. While a biosensor research study starts with an empirically grounded question and ends with an evidence-based conclusion, the intervening steps are often complex.
For students being exposed to the intricate nature of experimental design, data collection, and analysis techniques for the first time, it can be challenging to conceptualize the process as a whole.
Tying the conversation back to a model of the research study life-cycle can help to fill in procedural gaps that would normally only be understood after extensive practical experience conducting research studies.
Using a model such as the one depicted below provides a common reference frame for both the educator and student to orient lessons, exercises, and discussions. While simplifications are inevitable, a process map visualizes the directional, relational, and interlinking nature of various components along the way and fosters a systems-level understanding that ultimately leads to proactive thinking and planning when executing a research study.
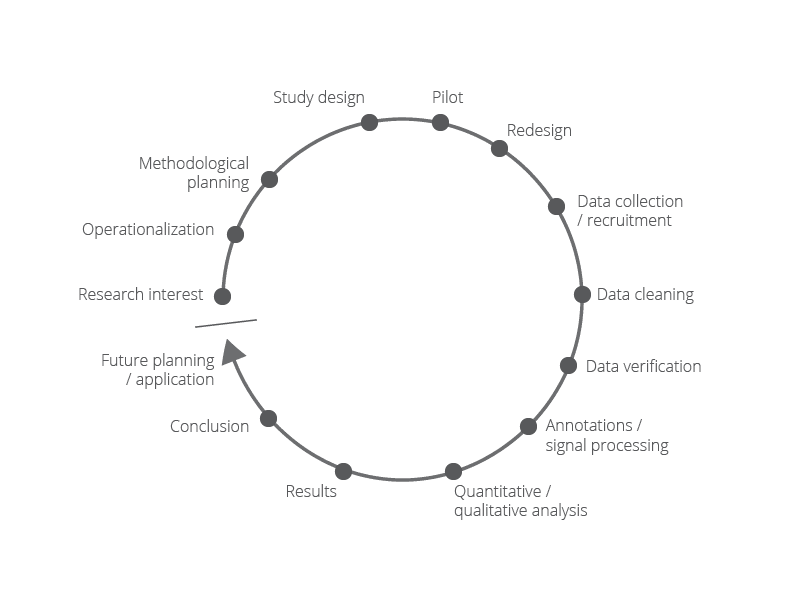
5. Reinforce values of scientific inquiry throughout
At the highest level, education in a scientific field develops a set of values for scientific inquiry which transcend any one particular topic or industry and follow students throughout their careers as analytical thinkers.
These values have been made explicit in the context of STEM education (Science, Technology, Engineering, Math) by the United States National Research Council [7]:
- Respect for the importance of logical thinking
- Precision
- Open-mindedness
- Objectivity
- Skepticism
- A requirement for transparent research procedures and honest reporting of findings
Cultivating a researcher’s mindset and familiarizing students with the ethical underpinnings of scientific research are equally important as training the use of sensors and data analysis techniques. Ideally, the importance of these values will become appreciated by students after hands-on experience with the scientific method.
However, an explicit review and discussion of each value can benefit even the most experienced of students. This review exercise will also serve illustrate the interconnected nature among the full set of values and the nature of a healthy system of scientific discourse.
Engage students with biosensor research and hands-on learning today! iMotions Learn products help students at the undergraduate level acquire both knowledge and skill within the field of human behavior research through biosensor data‐driven learning experiences. The topic‐specific content of each product provides a comprehensive overview of experimental theory as well as real‐world applications that emphasize the values embodied by scientific inquiry.
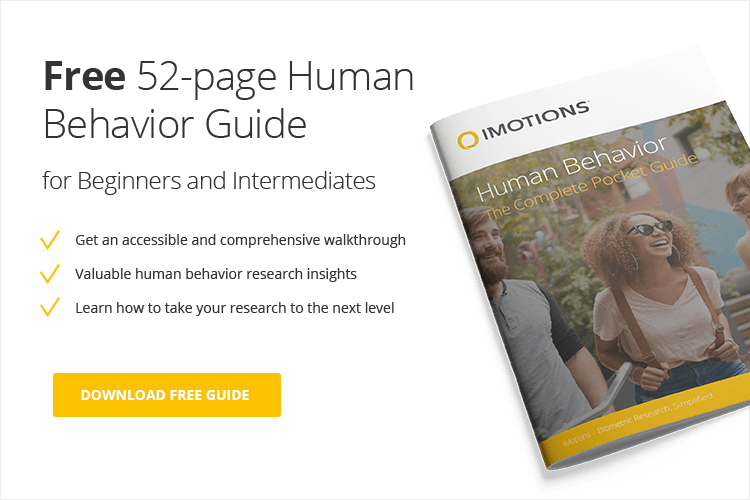
References
[1] “Work With New Electronic ‘Brains’ Opens Field For Army Math Experts”. The Hammond Times. p. 65. Retrieved May 20, 2019 – via Newspapers.com.
[2] Korwin, A. R., & Jones, R. E. (1990). Do hands-on, technology-based activities enhance learning by reinforcing cognitive knowledge and retention? Journal of Technology Education,1(2),39-50.
[3] Boucsein, W. (1992). Electrodermal activity. New York: Plenum.
[4] Fashler, S. R., Katz, J. (2014). More than meets the eye: visual attention biases in individuals reporting chronic pain. J Pain Res, 7:557–570.
[5] Deltomme, B., Mertens, G., Tibboel, H., & Braem, S. (2017). Instructed fear stimuli bias visual attention. Acta Psychologica. https://doi.org/10.1016/j.actpsy.2017.08.010
[6] Yang Z, Jackson T, Gao X, Chen H. (2012). Identifying selective visual attention biases related to fear of pain by tracking eye movements within a dot-probe paradigm. Pain, 153(8):1742-1748.
[7] Kennedy, T. J., & Odell, M. R. L. (2014). Engaging students in STEM education. Science Education International, 25(3), 246–258.