Table of Contents
- Introduction
- Infant development & health
- Infant health & developmental milestones
- History of infant research
- Use cases of infant research with biosensors
- Biosensors for infant tracking
- Technological advancements in infant biosensors
- Summary
- Challenges in infant biometric research
- How iMotions supports infant research
- The future of infant biometric research
- Conclusion
- Infant Research
- References
Introduction
This guide offers valuable insights into the foundational principles and recent advancements in infant research using biosensor technology. Whether you are an experienced researcher or new to the field, it provides useful information to help you enhance your research endeavors.
The guide begins with an overview of infant health and developmental milestones, followed by concrete use case examples of how researchers utilize biosensors to investigate topics ranging from parent-infant interactions to predicting sepsis. Next, it delves into the specific biosensors used in infant research, and explores the latest advancements in infant biosensor technologies.
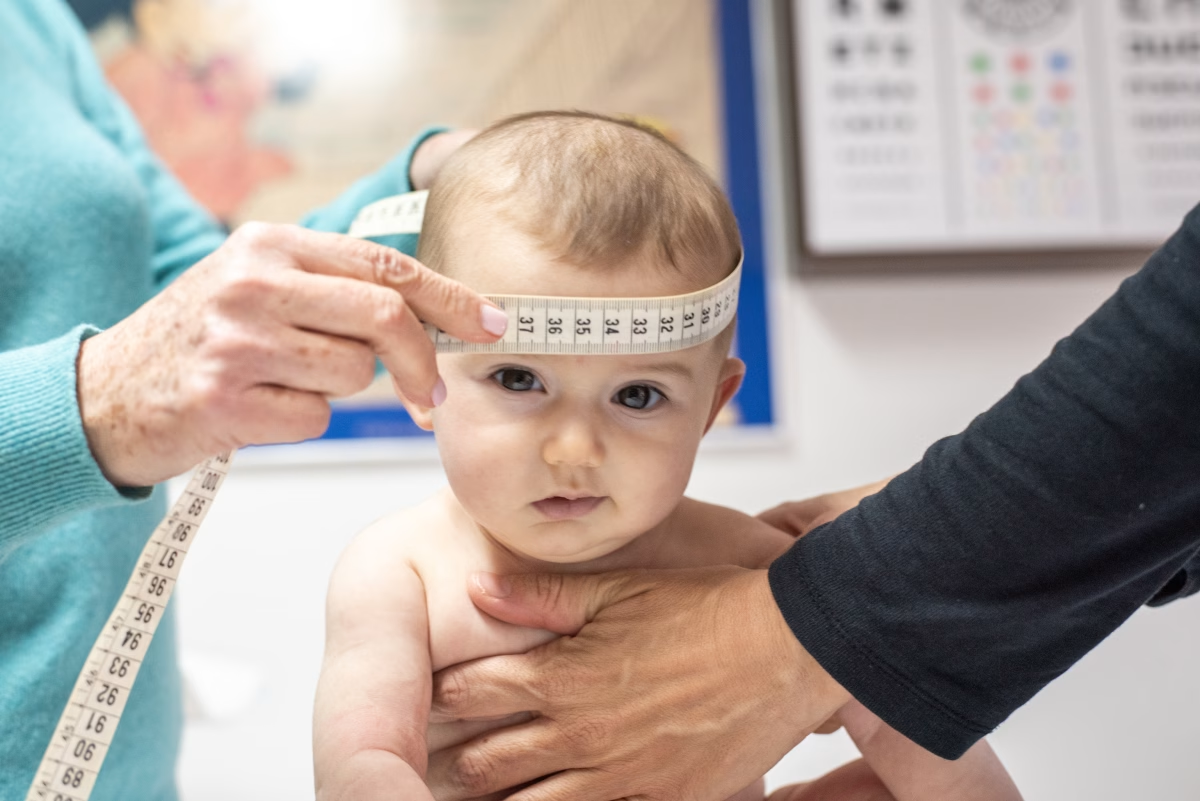
While infant research is rapidly growing, it still presents numerous challenges for researchers. This guide addresses the most common challenges in the field and introduces how iMotions offers solutions, serving as a platform for advancing infant research with cutting-edge biosensor technologies. We conclude with an overview of the future of infant biosensor research.
Our goal is to provide you with actionable steps to incorporate or advance biosensor technology in your infant research projects.
Infant development & health
According to the Center for Disease Control & Prevention (CDC), the term “infant” refers to a child in their first year of life (CDC). However, this is a loose definition and it is not uncommon for experts and the general public to use the terms infants when referring to children up to two years of age. After their first year of life, or when the child learns to walk, they have transitioned to toddlerhood. Table X provides an overview of what terms are used to describe the age group of young and unborn children.
Term | Age period |
Fetus | Throughout the gestational period, which typically lasts ~39-40 weeks |
Neonate | Day 0 – 28 after birth*Note: if born before gestation age (GA) 37 weeks the child is referred to as premature until it hits the GA 37 marker |
Infant | Day 0 – 12 months |
Toddler | 1 – 3 years |
Table X: Overview of the terms used to describe unborn and young children. Note that these terms are loosely defined and may not always be used as described here. Source: CDC, Wikipedia.
Infant health & developmental milestones
Infants undergo rapid development, leading to quick changes in their bodies and abilities. The following section offers an overview of the health markers and developmental milestones typically observed and measured in infant research.
Infant health markers
To evaluate, monitor and treat infant health, it’s important to know what their normal physiological values are. Compared to adults, infant measurements are substantially different. This table provides an overview of the average measures we can expect from an infant.
Infant | Adult | |
Resting, awake heart rate (beats per minute, bpm) | 0-1 months: 70-190 bpm1-11 months: 80-160 bpm (Kilinc & Snyder, 2020) | 60-100 bpm (note that well trained athletes may have a resting heart rate as a low as 40 bpm) (Levine, 2023) |
Heart rate variability (milliseconds, ms) | There is no clear consensus on the average HRV among infants*. (Latremouille et al., 2021; Oliveira et al., 2019, Mehta et al., 2022) | 42 ms, normal range: 19-75 ms (Lee, 2024; King, 2024) |
Blood pressure (Diastolic Blood pressure, mmHg) | Newborn: 64/41 mm Hg 1-12 months: 95/58 mm Hg (Mount Sinai, n.d.) | 120/80 mm Hg (CDC, 2024) |
Body temperature (celsius, C) | 0-12 months: 36.7-37.3 C (Healthline, 2024) | 37 C (Healthline, 2024) |
Respiration rate (breaths per minute, bpm) | 0-6 months: 30-60 bpm6-12 months: 30-50 bpm (Healthline, 2022; Reuter et al., 2014) | 12-20 bpm (Healthline, 2022) |
Table X: Overview of the average physiological measures in infants and adults. Note that the Heart Rate Variability (HRV) is reported as the Standard Deviation of all Normal to Normal RR (NN) intervals, the most commonly used time domain measure of HRV (Kleiger et al., 2005).
*Across studies, HRV results for infants vary (Claiborne et al., 2023; Latremouille et al., 2021; Chiera et al., 2020; Mehta et al., 2002). When you conduct your research it is important to be aware of these differences.
One of the major reasons for the difference between infant and adult health markers is that the autonomic nervous system (ANS), which controls the function of the heart and other vital organs, is not fully developed yet (Chiera et al., 2020). In fact, within the first 24 hours of an infant’s life, several ANS metrics change significantly, including heart rate and heart rate variability (Chiera et al., 2020).
The ANS continues to develop over the course of the first two years of life, contributing to major natural changes in physiological markers during this time period (Chiera et al., 2020). It is also important to keep in mind that infants are easily affected by their external environment, such that slight changes in temperature can have a measurable impact on their physiological activity (Chiera et al., 2020).
Infant developmental milestones
Many infants in wealthy nations now survive and avoid previously deadly diseases, thanks to medical advancements. This progress has shifted attention to other health issues such as sensory abnormalities (e.g., deafness), neurodevelopmental delays, and autism spectrum disorder. Understanding infant developmental milestones has empowered researchers and clinicians to detect developmental abnormalities early, allowing for timely intervention. The table below offers a general overview of the developmental milestones typically expected within the first year of a child’s life.
Types of development | Milestones (non-exhaustive) | References and further information |
Cognitive | Understands causality and object permanence | Malik and Marwaha, 2023; CDC’s Developmental Milestones | CDC |
Communication | Production of clear vowelsCanonical babbling, e.g. baba, mamaStarting to produce meaningful speechEnjoys being read toLooks or turns toward a new soundResponds to his or her nameImitation | Kuhl & Meltzoff, 1996;Age-Appropriate Speech and Hearing Milestones – Stanford Medicine Children’s Health |
Visual | Eye hand coordinationAbility to discriminate two objects within their visual field Depth perception | Infant Vision: Birth to 24 Months of Age | AOA |
Motor functions | CrawlingWalking (while holding on to furniture)Picks things up between fingersPulls up to stand | Adolph & Hoch, 2019; Adolph & Franchak, 2017 |
Table X: A non-exhaustive overview of an infant’s developmental milestones through their first 12 months of life
It is crucial to emphasize that during infancy, cognition, communication, and sensory abilities evolve rapidly. Take vocal development, for instance. Infants start with vocal cords more akin to non-human primates, but by six months, significant changes occur, leading to a vocal tract closer to adults (Kuhl & Meltzoff, 1996). This restructuring enhances motor control and expands vocal range. When researching infants and potential stimuli or interventions, it is vital to acknowledge these swift developmental shifts.
Another influential factor is environmental exposure and learning. Infants naturally mimic the sounds they hear, a process vital for acquiring language (Kuhl & Meltzoff, 1996). This means they adapt to their surroundings, ultimately sounding like “native speakers.” Thus, when studying infants, we must consider the profound impact of their external environment, which is inherently difficult to regulate.
History of infant research
As medical knowledge expanded during the 19th century, there was a growing recognition of the importance of specialized care for infants and children. Physicians began to grasp the significant differences in physiology, anatomy, and disease patterns between children and adults. This increasing concern for infant and child health and well-being culminated in the formation of the American Pediatric Society in 1888, which continues to play a prominent role in today’s pediatric research and healthcare communities. However, systematic and rigorous scientific investigations into infancy only gained momentum in the early to mid-20th century.
In the 1920s, psychologists began unveiling insights into the cognitive development of infants and young children. Notable figures such as Arnold Gesell, Jean Piaget, and Lev S. Vygotsky conducted continuous observation, careful monitoring, and behavioral experiments, revealing crucial insights into how infant, toddler, and child psychology unfolds. Similarly, in the mid-20th century, psychologists John Bowlby and Mary Ainsworth shed light on the establishment of mother-infant attachments and their enduring impact on a child’s health. Today, the research of these psychologists remains foundational in university teachings and informs much of the existing therapy and research frameworks.
The integration of biosensors into infant research is a relatively recent development, emerging in the late 20th and early 21st centuries. This integration has provided researchers with new tools to monitor various aspects of infant physiology and behavior more precisely and, in some cases, non-invasively. While early infant and child psychologists relied exclusively on observation and monitoring, the use of biosensors has expanded our understanding of infant development.
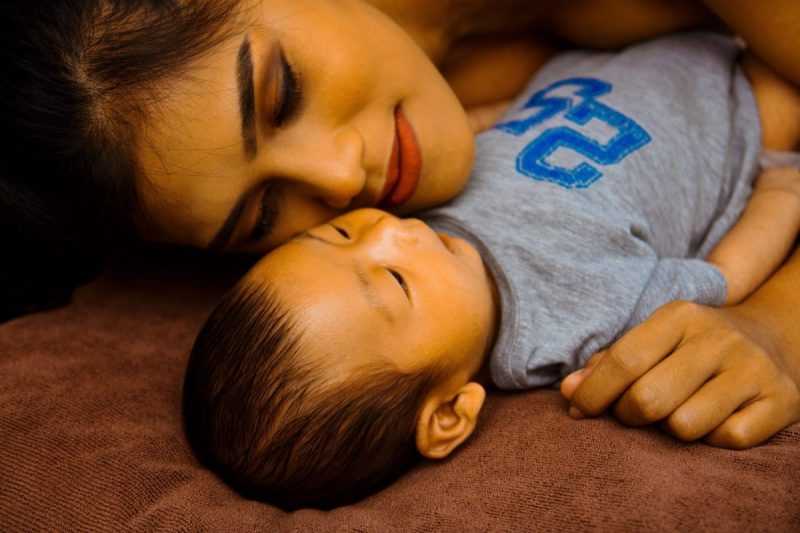
For example, biosensors can measure heart rate variability, respiratory patterns, sleep-wake cycles, body temperature, and even stress levels in infants. This information helps researchers investigate how these physiological parameters relate to developmental milestones, health outcomes, and environmental influences.
Moreover, biosensors have been incorporated into wearable devices designed specifically for infants, such as smart clothing and patches. These wearable biosensors enable continuous monitoring of infants’ vital signs and movement patterns, offering valuable insights into their health and development, both in clinical settings and at home. Overall, the integration of biosensors into infant research represents a significant advancement, offering researchers new opportunities to explore infant physiology, behavior, and development with greater precision and depth.
Use cases of infant research with biosensors
Current researchers investigate a broad range of infant topics ranging from infectious diseases, to cognitive development to parent-infant attachment styles. Below, we provide a brief literature review of select infant research use cases. Please refer to table X for insights on further infant research topics using biosensors.
Predicting and detecting Sepsis and Sudden Infant Death Syndrome (SIDS)
Sepsis is a bacterial infection that can lead to organ failure, while Sudden Infant Death Syndrome (SIDS) is the unexplained death, usually during sleep, of a baby younger than 1 year old. Death from sepsis and SIDS continue to remain high, with SIDS being particularly unpredictable. Researchers have utilized biosensors to monitor infants in the hospital and at home to detect early signs of SIDS or distress from sepsis (Chiera et al., 2020; Kumar et al., 2020).
In the case of SIDS, studies have found that hours before SIDS occurs, an infant experiences a measurable drop in their heart rate and their heart rate variability (HRV). Using video-based PPG (Photoplethysmography, a method to measure blood volume changes in the skin), a contact-less, and in some cases even remote, sensor, scientists are now able to continuously monitor an infant’s heart rate in real time to detect early signs of SIDS (Zhao et al., 2016; Zhao et al., 2013).
Similarly, a study utilizing heart rate, movement and respiration monitoring, identified significant changes during the 24 hours prior to the diagnosis of late-onset sepsis (Joshi et al., 2020). In particular during the last 6 hours, the researchers identified marked heart rate deceleration, increased respiratory instability, and a reduction in spontaneous movement.
Because these changes in infant vital signs can be undetectable to the human eye as well as to traditional Neonatal Intensive Care Unit (NICU) monitoring tools, biosensor systems are proving to be a life-saving resource (Fairchild and O’Shea, 2010).
Detecting early biomarkers of Autism Spectrum Disorders
According to the Center for Disease Control (CDC), it is estimated that 1 in 6 children are diagnosed with a neurodevelopmental disorder, and 1 in 36 children in the US experience Autism Spectrum Disorder (ASD). While all racial, ethnic and sociodemographic groups can experience ASD, boys are four times more likely than girls to be affected. ASD and other neurodevelopmental disorders, such as attention deficit hyperactivity disorder (ADHD), have undergone a dramatic rise during the past quarter century.
While it is well-establish that early intervention, before the first 2-3 years of life (National Institute of Child Health and Human Development, 2021), is effective in improving symptom management and life quality, ASD is typically not diagnosed until they are 5 years old (Maenner et al., 2023). Thus, to close this gap, increasing research is focused on detecting early biomarkers of ASD.
In one such study, researchers tested the eye gaze patterns of sixty four 10 month old infants, 47 of whom were at high risk of developing ASD due to having at least one older sibling with the diagnosis (Thorup et al., 2016). Each infant, seated on the lap of their parents, sat in front of an eye-tracker that could follow where the infant was looking. They found that the infants in the high risk group displayed reduced performance in gaze following compared to low-risk infants (Thorup et al., 2016). In a similar study with almost 1500 toddlers (mean age 2 years), researchers found that infants with symptoms of ASD displayed abnormal gaze fixation patterns (Wen et al., 2022).
Moreover, this correlated with their clinical profile (Wen et al., 2022). Studies have even shown that gaze behavior recorded at 4 months of age using eye tracking predicts cognitive function at age 3 (Kaul et al., 2016). It is well known that gaze patterns are altered in individuals with ASD, but the majority of this data is collected during the teenage years or adulthood (see for example Dalton et al., 2005 , Klin et al., 2002 and Ness et al., 2017). Therefore, the research described above represents a critical advancement for early intervention.
While eye-tracking is one of the most popular technologies to measure infant and child cognitive development, other sensors are also effective. Often, other sensors are also more convenient than eye tracking devices. One study developed an app that could track facial and gaze movements, head position, and task performance through touch sensors and voice detection in toddlers between 17-36 months (Perochon et al., 2023). From these features, machine learning algorithms inferred with a high level of accuracy if the child was likely to have an ASD diagnosis (Area Under the Curve (AUC) = 0.90; meaning the algorithm correctly distinguishes between children with and without ASD 90% of the time).
This sort of technology presents a novel advancement in accessing and distributing diagnostic support to a broader population that normally would not or could not participate in in-person research studies.. Moreover, an often overlooked challenge is that many traditional research studies have not included girls and children of color, hampering the ability of biometric studies to accurately measure ASD risk in these populations. Perochon et al.’s algorithm was capable of detecting ASD status accurately in all populations of toddlers regardless of race or sex (Perochon et al., 2023).
Understanding parent-infant dynamics
Since the 1950s, researchers have studied the quality, factors and long-term effects of parent-infant dynamics. While the majority of studies have focused on the mother-infant relationship, more studies are starting to investigate the father-infant relationship as well.
Behavioral coding (the detailed analysis of behavior patterns) through video and live observation are powerful, but labor intensive, tools to understand the parent-infant dynamics. With the advent of biosensor tools, technology has started supplementing behavioral coding while also reducing labor intensiveness. Using head-mounted eye tracking glasses, a small but longitudinal study of five parent-infant dyads (infants between 10-15 months old), researchers identified factors modifying eye contact and gaze communication in their home environment (Yamamoto et al., 2019).
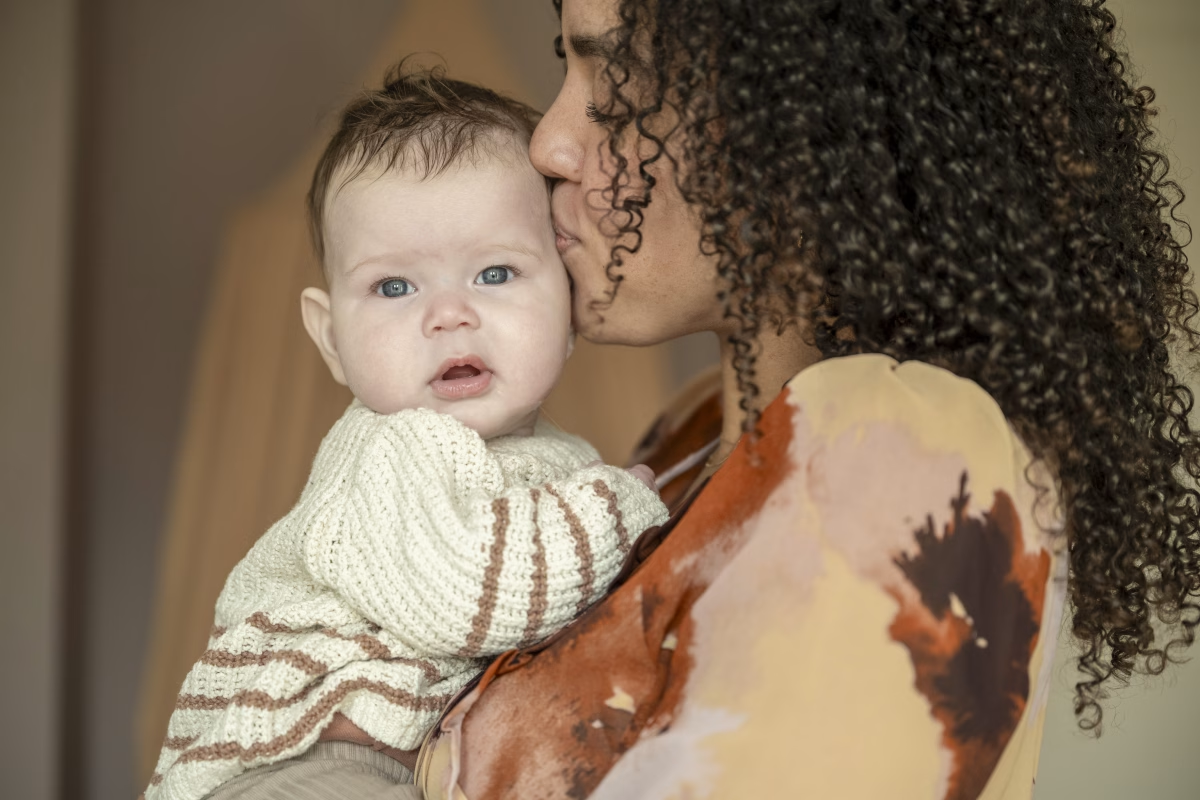
Another study identified unique brain correlates between infants and their mothers using dual electroencephalogram (EEG) recordings (Endevelt-Shapira and Feldman, 2023). In this innovative study, researchers measured the correlations in brain activity between the mothers and their 5-12 month old infants during face-to-face interactions. The researchers found that higher maternal sensitivity correlated positively with greater mother-infant brain synchrony. In contrast, when mothers scored high on maternal intrusiveness, the researchers measured lower inter-brain coordination.
Traditionally, attachment studies investigated how infant stress affected the mother-infant dyad. A new study from 2021, explored the opposite relationship: what happens to the infant’s autonomic nervous system when their mother is in distress (Mueller et al., 2021) ? The study found that after an ecological stressor that only affected the mother, the infant’s heart rate and respiratory sinus arrhythmia (a measure to study parasympathetic nervous system activity) were negatively affected.
This study suggested that maternal stress is “picked up” by the infant, even if the infant was not a direct recipient of the stress. A subsequent dyadic stressor (known as The Still Face Paradigm), led to a further exacerbation of ANS dysregulation in infants whose mothers had been exposed to a stressor. This suggests that when mothers are already stressed, it becomes harder for them and their infants to regulate their emotional and physiological state. This process, also known as co-regulation is critical for long-term, healthy ANS development in infants.
A note on the use of biosensors in these case studies
In the majority of the case studies discussed above, they often only employ one – or maybe two – biosensors, with widespread usage of eye tracking, heart rate sensors, and respiratory sensors. In more advanced studies, methods like EEG are not uncommon to find. However, as demonstrated by Perochon et al., 2023, infant tracking does not have to be limited to this small number of biosensors, nor does it have to involve sensors that directly contact the body.
Technological advances enabling voice analysis and video-based facial expression analysis are starting to be employed in infant research as well. A limiting factor in applying these contactless technologies to infants is the lack of datasets that accurately validate the metrics, as existing databases primarily consist of adult data (e.g adult faces or adult voices) (Zaharieva et al., 2024; Onal Ertugrul et al., 2022). However, research groups are starting to change this and new studies have demonstrated possibilities of detecting infant facial expressions as well as voice characteristics (Onal Ertugrul et al., 2022; Li et al., 2021).
Newer studies are prioritizing developing multimodal biometric insights into infant behavior by using a combination of simultaneous methods, such as behavior coding, EEG, heart rate monitoring, movement sensing, and respiration sensing (Islam et al., 2024; Chen et al., 2023). More ingenious approaches are integrating sensors into “smart clothes” where sensors are integrated into the clothing the child is wearing (see for example: Islam et al., 2024 and Airaksinen et al., 2020). It is clear that the infant biosensor technology landscape is changing rapidly with remarkable potentials for novel ways of understanding infant behavior.
Biosensors for infant tracking
In this section we will dive into the biosensors that are currently available and being used for infant tracking. It is important to note that these sensors undergo constant development, continuously changing the biosensor landscape available for infant research.
We will start by providing a brief overview of the different biosensors currently being used for infant tracking, followed by a section exploring the active advances in infant biosensors that we expect will become the “new normal” in the coming years.
Biosensors used in infant research
The table below provides an overview of what biosensors can be used to measure distinct infant behaviors and physiologies,together with example use cases, and relevant references you can explore to learn more. In the following, we will dive into a subset of these sensors.
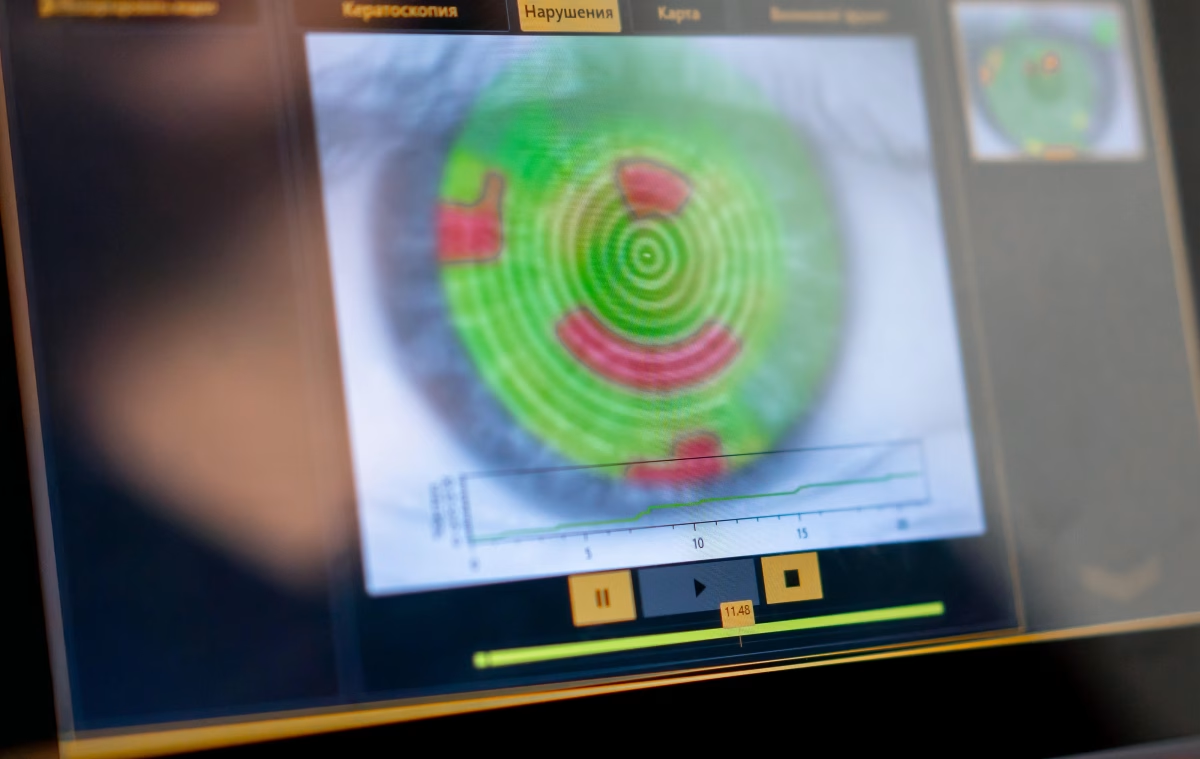
*Galvanic skin response (GSR) is also known as electrodermal activity (EDA).
Measurement objective | Biosensor technology options | Example use cases | Relevant references |
Body movement | Video Observation & Behavioral CodingAccelerometryInertial movement units (IMUs)Pressure sensor | Seizure detection, monitoring and interventionMotor function developmentAgencySleep behaviorNeurodevelopmental disorder detection, monitoring and intervention | Chen et al., 2023; Sloan et al., 2023; Airaksinen et al., 2020; Crespo-Llado et al., 2018; Chen et al., 2016; |
Heart rate | Electrocardiography (ECG)Photoplethysmography (PPG)Video PPG (vPPG)BallistrographyCardiac acoustics | Sudden infant death syndrome (SIDS)SepsisParent-Infant interactions and attachmentsSleep behaviorSensory development Stress reactivity | Weiss et al., 2024; Chen et al., 2023; Mueller et al., 2021; Joshi et al., 2020; Chiera et al., 2020; Zuzarte et al., 2019; Zhao et al., 2016; Richard and Mosjo, 2004; Schechtman et al., 1988; Harper et al., 1976 |
Blood oxygenation | Pulse oximetry | Heart disease detectionRespiratory function and developmentSleep apnea detection | Govindaswami et al., 2012; Carbone et al., 1999; Loscher et al., 1990 |
Respiration | Pulse oximetryBarometric pressure sensorsPiezoelectric sensorRespiratory Inductive plethysmography (RIP) | Apnea and asthma detectionSIDSSepsisStress reactivity Seizure detection | Chen et al., 2023; Joshi et al., 2020; Rash et al., 2016; Bosquet Enlow et al., 2014; Ritz et al., 2012 |
Voice analysis | Manual scoringAutomatic audio feature extraction and classification platforms, e.g. OpenSmile | Infant cry analysis Pathology detection, e.g. asphyxia, hypo-acoustic (hearing disorder), cleft palate and hypothyroidism. Parent-infant communication | Yasin et al., 2022; Ji et al., 2021; Li et al., 2021; Levrero et al., 2018; Kult & Meltzoff, 1996 |
Facial expression | Video monitoring and manual facial coding (Baby FACS)Camera-based facial coding | Visual attentionEmotion regulationNonverbal communicationParent-Infant interactions | Zaharieva et al., 2024; Onal Ertugrul et al., 2022; Crespo-Llado et al., 2018; Rayson et al., 2017; Izard et al., 1995 |
Skin conductance | Galvanic skin response (GSR)* | PainInfant temperamentArousalAutonomic nervous system development | Visnovcova et al., 2022; Buthman et al., 2018; Ham & Tronick, 2009; Eriksson et al., 2008; Storm, 1999 |
Eye tracking | Infrared eye tracking technologyScreen-basedHead MountedWebcam-based eye tracking | Cognitive and visual developmentNeurodevelopmental disordersParent-infant interactions | Borjon et al., 2021; Leppanen et al., 2021; Yamamoto et al., 2019; Kaul et al., 2016; Thorup et al., 2016 |
Brain activity | Electroencephalogram (EEG)Functional near-infrared spectrometry (fNIRS) | Cognitive developmentEarly identification of neurodevelopmental disorderSeizure detection, monitoring and interventionSleep behavior | Gervain et al., 2023; Filippa et al., 2023; Endevelt-Shapari & Feldman, 2023; Nayak et al., 2023; Borjon et al., 2021; Weibley et al., 2021; Dzwilewski et al., 2020; Jones et al., 2020; Crespo-Llado et al., 2018; Briton et al., 2016; Lloyd-Fox et al., 2014 |
Table X: This table provides an overview of the most common biosensors currently used for infant research.
Accelerometer sensors
Accelerometers and gyroscopes have been used in pediatric research since 2010, but their application has become more widespread over the past decade. In most studies, these accelerometers are worn around the infant’s wrist or ankle and may need to be left in place for several days to obtain reliable wake and sedentary activity measures, depending on the research question (Ricardo et al., 2018; Pitchford et al., 2017). Researchers use accelerometers to study infant sleep patterns, behaviors, and motor development. Notably, studies have found that accelerometers provide more accurate data than parent recall on the amount of time an infant spends in various motor activities. This accuracy is crucial for informing clinical decisions. (Manning et al., 2023).
Video observation and behavioral coding
One of the most commonly used and traditional methods for capturing infant behavior is behavioral coding through video observation. Whether documenting facial expressions, motor movements, or sleep patterns, video observation followed by manual coding is considered a highly reliable approach (Zaharieva et al., 2024; Crespo-Llado et al., 2018; Rayson et al., 2017). The major drawback of behavioral coding is its time-consuming nature. However, for certain behaviors, such as facial expressions, there are currently no well-validated technological alternatives for infants. As a result, manual coding using the FACS method remains the gold standard (Forestell and Mennella, 2012; Ekman and Friesen, 1978).
Electrocardiography (ECG) & Photoplethysmography (PPG) sensors
Measuring heart rate is a core component of many infant research studies (Chiera et al., 2020). By far, ECG and PPG are the most commonly used methods, each with distinct advantages and disadvantages. ECG is considered the gold standard for measuring heart rate, but its “clunkier” setup can hinder natural infant movements. In contrast, PPG measures heart rate through changes in arterial volume, offering easier sensor placement with minimal disruption to behavior.
However, PPG sensors are more susceptible to movement artifacts compared to the robustness of ECG sensors (Tamura et al., 2024; Chiera et al., 2020). With that said, technological advances have been made to reduce PPG’s movement sensitivity (Sahni et al., 2003), and PPG is an integral part of most wearable devices offered today.
In recent years a new method of capturing heart rate has emerged: video based PPG (vPPG). vPPG utilizes contactless optical technology to remotely detect blood volume changes through skin blood perfusion (Tamura et al., 2024; Chiera et al., 2020). Under natural light conditions, consumer cameras can capture the minute changes in skin color caused by variations in blood flow.
This technology allows researchers to derive heart rate without attaching any device to the infant’s body, and has already been tested for its potential in preventing SIDS (Zhao et al., 2016). It is important to mention that changes in lighting conditions can affect signal quality, and ongoing studies are investigating the implementation of vPPG under different lighting conditions (Cobos-Torres et al., 2018).
Eye-tracking sensors
Eye tracking of the infant gaze has been reported since the 1930’s, but it is not since the last couple of decades that the implementation of eye tracking for infant research has become widespread (Aslin, 2012). Just as for adults, many factors must be taken into consideration when conducting eye tracking research with infants. Should the eye tracking device be head mounted or screen based? What sampling rate do we need? Will we track fixations or saccades? What sort of visual stimuli can we present and expect to track? The answers to these questions will depend entirely on your research goal, however, there are a few pointers to keep in mind:
- In general, eye tracking studies with infants utilize eye trackers with sampling rate ranging from 30-300 Hz (Aslin, 2012; Corbetta et al., 2012).
- Because the infant visual system is not fully developed, it is more difficult to obtain a high-accuracy calibration. Experts suggest that you should not expect eye tracking accuracy better than 1 degree of visual angle (dva) when working with an infant, and your research question should be informed accordingly (Aslin, 2012).
- The visual stimuli and task must be designed with infant age and visual and cognitive development in mind. For example, 2 month old infants will perform very differently on an object recognition task compared to a 6 month old. Gredeback et al. provides an excellent review of eye tracking study designs for infant research (Gredeback et al., 2010).
You can learn more about eye tracking in our pocket guide on eye tracking research.
Electroencephalogram (EEG) sensors
EEG is notoriously challenging to work with, primarily due to its complex and time-consuming setup, as well as its substantial data processing requirements. However, setting up EEG in infants is somewhat easier because they typically have little to no hair and their skin is very thin (Britton et al., 2016). These factors improve the impedance which enhances the signal quality of the EEG electrodes.
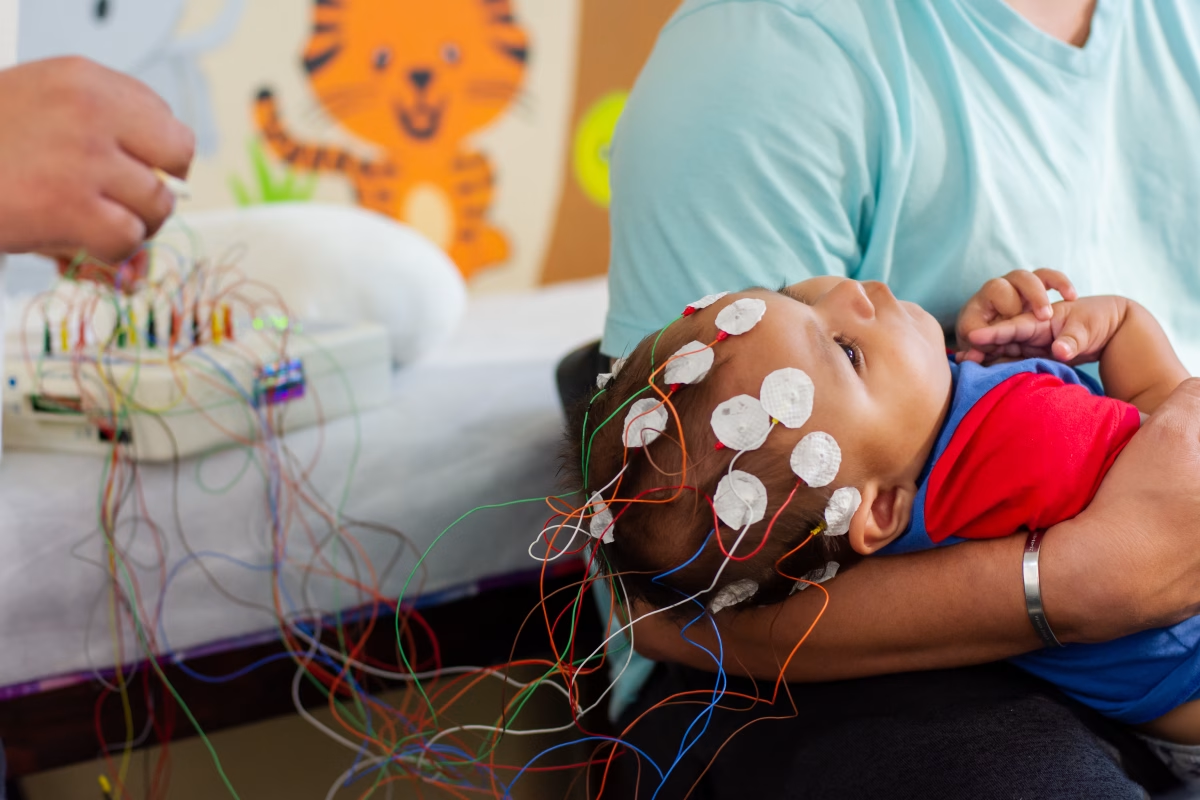
However, one must still consider the movement artifacts and discomfort caused to the infant (Britton et al., 2016). Similar to the ANS, visual system, and sensorimotor system, brain activity patterns undergo rapid changes throughout an infant’s development. Consequently, varying types of baseline activity are recorded at different developmental stages, and their brain responses to stimuli also vary with age.
Chetan and Anikumar provide useful insights into the methods and steps to consider when conducting infant EEG research (Nayak and Anikumar, 2024). While the invasiveness of EEG sensors makes it less attractive for infant research, it is a very powerful biosensor with the ability to predict cognitive and neurodevelopment (Jones et al., 2020).
Technological advancements in infant biosensors
Since the early 20th and 21st centuries, when biosensors were first incorporated into infant research, significant technological advancements have been achieved. While it is impossible to cover all these developments in this section, a summary would likely highlight the following key terms: stretchable, wireless, and contactless. In the following sections, we will provide examples of each of these major advancements.
Stretchable electronic patches
Biosensors for adults can often be cumbersome, and for infants, they can hinder natural movement. A significant advancement in this field is the development of sensors integrated into a simple, stretchable electronic patch (Zhou et al., 2024; Kim et al., 2023; Chen et al., 2016). These patches can be effortlessly applied to an infant’s body without restricting their movements. Directly adhering to the skin, these patches can monitor vital signs such as heart rate, respiration, and temperature. They are proving to be both cost-effective and user-friendly, making them suitable for use in hospitals as well as for home monitoring (Xu et al., 2021).
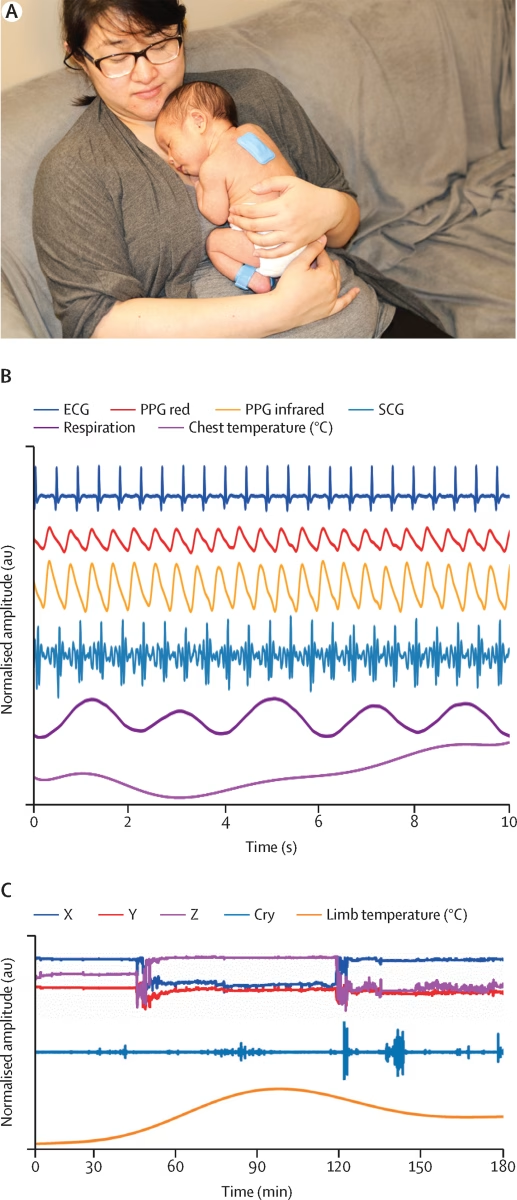
Wireless biosensors
Extensive research and monitoring are conducted on preterm infants during their time in the neonatal intensive care unit (NICU). Traditionally, these infants are continuously monitored using devices attached to their bodies, such as sensors for heart rate and temperature. These sensors are linked to nearby machines, creating challenges when moving the infant in and out of the incubator, as well as during interactions with nurses or parents (Chung et al., 2020).
To address this issue, an increasing number of infant-suitable sensors are now wireless (see Memon et al., 2020, for an excellent overview of wireless sensor options for infants). These options range from stretchable electronic patches (as discussed earlier) to smart clothing and wristbands. Synchronizing different sensors placed on the infant’s body simultaneously allows for more detailed data analysis and feature extraction (Chung et al., 2019).
Contactless biosensors
Contactless biosensors, as the name suggests, do not require direct physical contact. Utilizing video or microphones, these sensors can capture meaningful changes in infant behaviors. Traditionally, video observation of infant behaviors has been a standard approach in research. However, newer technologies, such as video-based photoplethysmography (PPG), can extract pulse and heart rate in a completely contactless and even remote manner (Tamura et al., 2024; Chiera et al., 2020).
Another advanced contactless biosensor is video-based, online eye tracking. While this new technology is increasingly tested and well-established in adult research, it is still emerging in infant research due to the unique aspects of infant eye anatomy and function. Despite this, video-based, online eye tracking holds significant potential for advancing infant research (Banki et al., 2022).
Summary
In summary, a wide range of biosensors is currently available for infant research, and the choice of sensor depends on the specific behavior and developmental stage you aim to study. The field is continually evolving, with efforts focused on reducing hardware complexity, simplifying setup, and enhancing user-friendliness.
Challenges in infant biometric research
While infant research is exciting, it also presents significant challenges. In the following we will provide an overview of four challenges to be aware of.
Rapid development
Infancy is a period of rapid and unique motor, brain, and sensory development. While this is an exciting time for researchers, it also presents challenges in capturing meaningful changes across different time points and groups of infants. To address these challenges, researchers can employ within-subject designs, measuring differences or changes in behavior within the same infant over multiple time points. This approach helps to account for individual variability.
Alternatively, between-subject designs, which compare different groups of infants, can address these challenges by increasing the sample size and allowing for broader generalizations. Both methods are valuable for gaining comprehensive insights into infant development, effectively addressing the challenges posed by the rapid and unique developmental changes each infant undergoes.
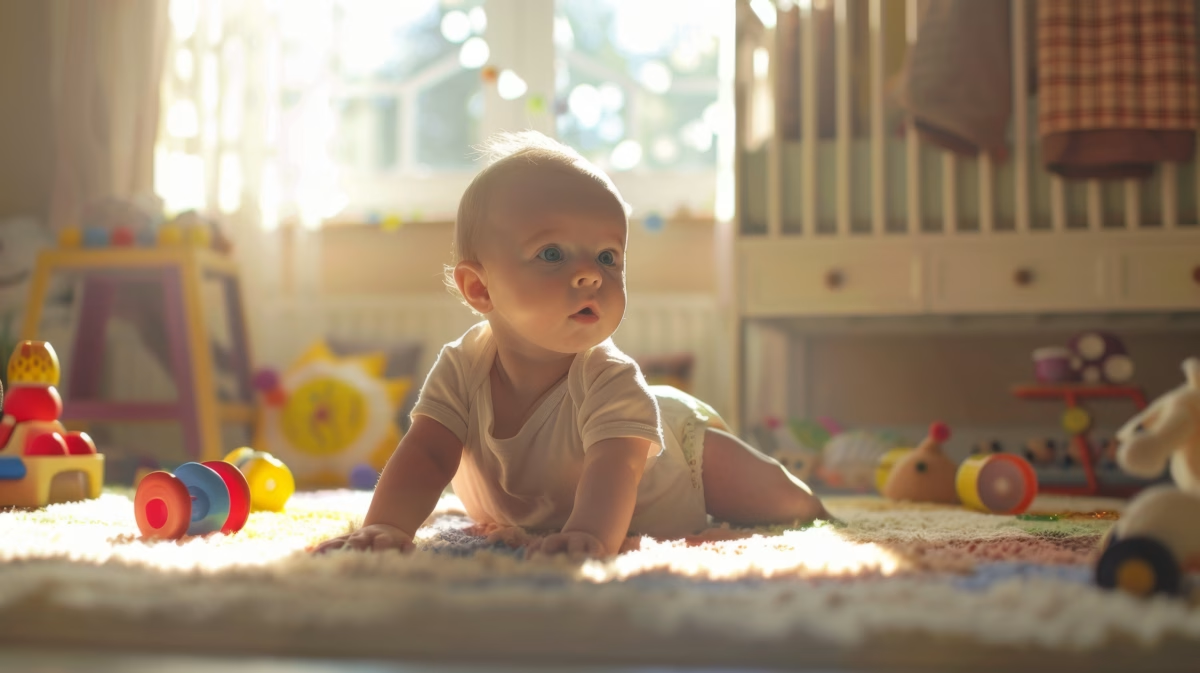
Limited communication and environmental factors
Limited verbal communication with infants makes it challenging to accurately assess their needs, discomfort, and responses, forcing both parents and researchers to rely on indirect measures. This reliance can complicate the validity and reliability of interpretations. Additionally, environmental and household factors, such as parental care, temperature, and noise levels, significantly impact an infant’s long-term development and immediate behavior. These variables add further complexity to the interpretation of behavioral, physiological, and psychological data in research.
Technological limitations
While this pocket guide has presented numerous options for infant research tools, obtaining high-quality, user-friendly technology for infant research can be challenging and expensive. Researchers often need to evaluate offers from multiple vendors and invest more money to access the necessary technology.
Complex data analysis
While we can encourage specific behaviors in infants, such as using engaging calibration dots for eye-tracking research or making playful faces to elicit vocalizations, infants are unlikely to follow instructions and almost certain not to remain still. As a result, our data may be less well-calibrated and more prone to movement artifacts. This introduces additional complexity to data analysis, which can be both time-consuming and costly. Seeking support from professionals experienced in infant research for data analysis can prove to be a valuable investment for your project.
How iMotions supports infant research
iMotions’ advanced multimodal and behavioral coding platform guarantees a seamless experience for conducting cutting-edge infant research. Below, we detail key aspects of the iMotions platform that specifically enhance studies involving infants.
Infant Behavioral coding
While iMotions prides itself on enabling seamless multimodal biosensor recordings, the platform is also a robust solution for behavioral coding. Researchers can utilize iMotions’ annotation and marker tools to pinpoint specific moments and durations of infant behavior. These tools support both live observations and post-recording analysis, with the annotation tool being particularly effective for marking extended periods of behaviors. These tools are particularly effective for facial expression and movement analysis.
Recently, iMotions introduced automatic markers that timestamp biosensor events. This feature allows researchers to easily correlate behavioral events, such as a smile or head movement, with corresponding changes in biosensor measurements, enhancing the precision of their studies.
Multi-stream video capture
iMotions can capture video streams from three video feeds simultaneously, providing researchers with the ability to observe and analyze minute movements across the infant’s entire body and face. Technically, it is possible to split the video feeds with hardware or software tools like ManyCam and Logitech to have four feeds to show up as one camera. It is important to note that this approach would work well for capturing unique body parts and movements, but not multiple faces for facial expression analysis due to video restrictions by Affdex, the software used to analyze facial expressions.
This multi-stream capture capability is essential for detailed behavioral coding and analysis and is utilized heavily in current research publications. Furthermore, with advancements in video-based photoplethysmography (PPG) and respiration monitoring, these video streams are increasingly valuable as non-contact biosensor measures.
Data capture of multimodal biosensors
iMotions can simultaneously capture data from multiple biosensors, limited only by the capabilities of your computer and network. The trend among advanced research groups is increasingly towards a multimodal biosensor approach in infant studies. This approach underscores the importance of considering multiple metrics to fully comprehend infant behavior, much like adult behavior.
For instance, understanding whether heightened arousal measured by galvanic skin response (GSR) corresponds to a positive or negative event requires integrating GSR data with facial expression analysis (FEA). iMotions’ API and LSL integration empower users to seamlessly integrate virtually any type of biosensor into their data collection process.
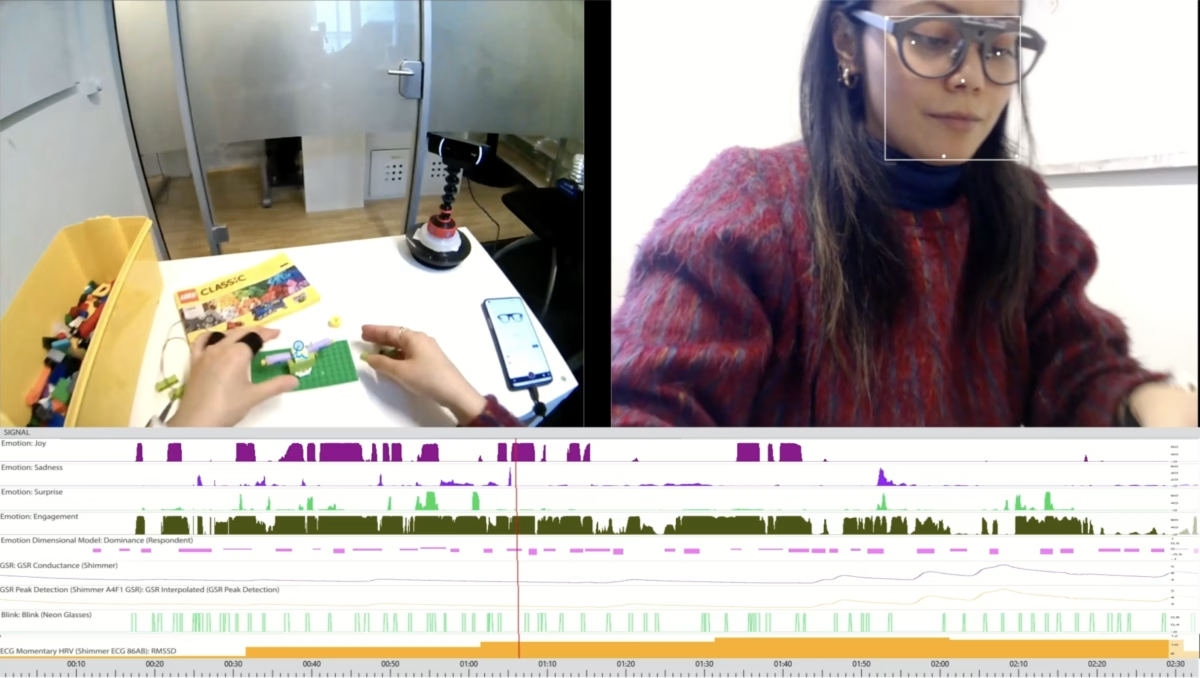
Infant eye tracking
iMotions integrates with numerous high-quality eye tracking hardware providers, including Smart Eye, Pupil Labs, Argus Science, and Eye Tech. Furthermore, through iMotions’ generic eye tracking data importer, data gathered with Positive Science eye-tracking glasses, a commonly used brand for infant and toddler research, can be imported. These hardwares offer a wide range of sample rate options, ranging from 30 Hz-250 Hz; representing the spectrum of sampling rates most commonly used in infant research.
When conducting infant eye-tracking research, two common challenges often arise:
- Infant Movement: Infants naturally move their heads during sample collection since they cannot follow spoken instructions. Screen-based eye trackers rely on a “headbox,” the spatial area where they can detect and record eye movements. Some eye trackers have very small headboxes, requiring minimal movement from the subject to obtain high-quality data. For infant research, it is crucial to choose an eye tracking system with a larger headbox to accommodate the infant’s natural head movements while still capturing high-quality data.
- Parental Presence: In modern laboratory settings, infants are often seated on a parent’s lap during eye-tracking studies. This setup can introduce inaccuracies as the eye tracker may mistakenly track the parent’s eyes instead of the infant’s. If this is your setup, consider using an eye tracker that can differentiate between subjects or opt for eye tracking glasses to ensure accurate data collection.
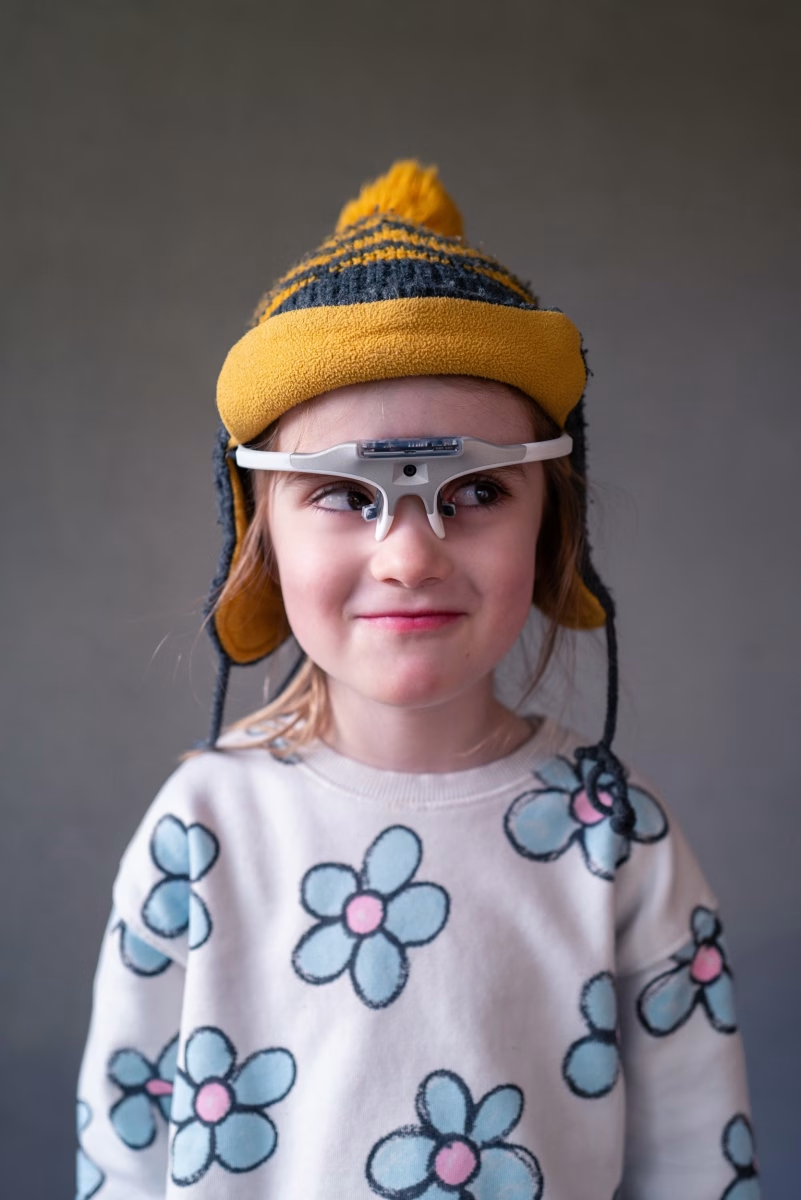
In either scenario, iMotions can provide recommendations on the eye tracking hardware that best supports your experimental needs.
Data analysis and Exports
Data analysis can be time consuming and labor intensive. iMotions simplifies that process by providing access to automated R scripts that calculate the most common metrics derived from a variety of biosensor technologies, for example heart rate variability, respiration rate, fixation duration, and EEG power spectrums. You can receive this data in a variety of formats, including:
- Data files with the unprocessed sensor data
- Data files with filtered and analyzed sensor data
- Emotional heatmap: a visualization and quantification of how eye tracking and facial expressions interact
- Methods and graphics with summary statistics
These analysis and export opportunities address a wide range of needs that different research projects may entail.
The future of infant biometric research
The future of infant research holds promise with advancements in technology and methodologies that enhance our understanding of early development. Here are some key aspects shaping the future:
Multimodal Approaches
There is an increasing focus on using multiple types of data simultaneously, such as biosensors, video analysis, and physiological measures, to obtain a comprehensive understanding of infant behavior and development. This multimodal approach enables researchers to investigate complex interactions between physiological responses, behaviors, and environmental stimuli.
Engineering researchers are further advancing this field by integrating multimodal biosensor technology into smart clothing for infants, such as suits, shoes, and swaddles (Airaksinen et al., 2022; Airaksinen et al., 2020; Memon et al., 2020; Zhu et al., 2015). Additionally, pressure-sensing mats have been developed to capture infant movements (Kulvicius et al., 2023). These innovations represent significant strides in our ability to monitor and measure infant behavior in naturalistic settings, beyond the confines of the laboratory.
Non-invasive and Contactless Monitoring
Technologies such as video-based analyses for measuring physiological responses (e.g., heart rate, respiration, posture) without physical contact are becoming increasingly sophisticated. These methods minimize disturbances to infants during data collection, enabling more naturalistic observations. Remarkably, these measurements can now be captured using a smartphone (Bae et al., 2022; Valentine et al., 2022; Liu et al., 2021), including in infants (Chung et al., 2023). While further research is needed, this advancement highlights the increasing capability to conduct infant research outside the lab and in natural environments.
Big Data and Machine Learning
As data collection becomes more sophisticated, there is a growing emphasis on leveraging big data techniques and machine learning algorithms to analyze large datasets. With the rise of multimodal data collection, advanced data analysis methods are continually evolving. These innovations allow researchers to identify subtle patterns and correlations that traditional methods may overlook, enhancing our understanding of infant behavior and development.
Longitudinal Studies
As sensors become easier to integrate into daily life, the number of longitudinal studies tracking infants from birth through childhood and beyond is expected to rise. These studies will provide valuable insights into typical and atypical development, individual differences, and the impact of early life experiences on later outcomes. Integrating sensors into clothing represents an innovative approach to achieving this goal.
Cross-disciplinary Collaboration
Advanced infant research increasingly relies on the combined expertise of psychologists, neuroscientists, engineers, and data analysts. As the field continues to grow, we can expect more interdisciplinary collaborations to generate the most innovative and insightful findings.
Conclusion
In this pocket guide, we delved into the use of biosensor technology in infant research. While infant research began in the early 20th century, the incorporation of biosensors only became widespread in the past few decades. Despite this delay, the use of biosensor tools in infant research has rapidly expanded. Today, measuring an infant’s heart rate, respiration, and temperature is routine in many research departments as well as in the Neonatal Intensive Care Units.
However, applying hardware to small infants can still be challenging. Advances in technology are addressing this by integrating biosensors into infant clothing and diapers, and reducing hardware to sticky, stretchable patches. These innovations are revolutionizing how we study infants both in the lab and in natural settings.
iMotions is dedicated to advancing infant research with cutting-edge biosensor tools. We continually develop solutions that provide validated and user-friendly insights into infant behavior and physiology, so that your research can have even more impact.
Infant Research
The Complete Pocket Guide
- 32 pages of comprehensive material on infant research
- Valuable infant research insights (with examples)
- Learn how to take your research to the next level
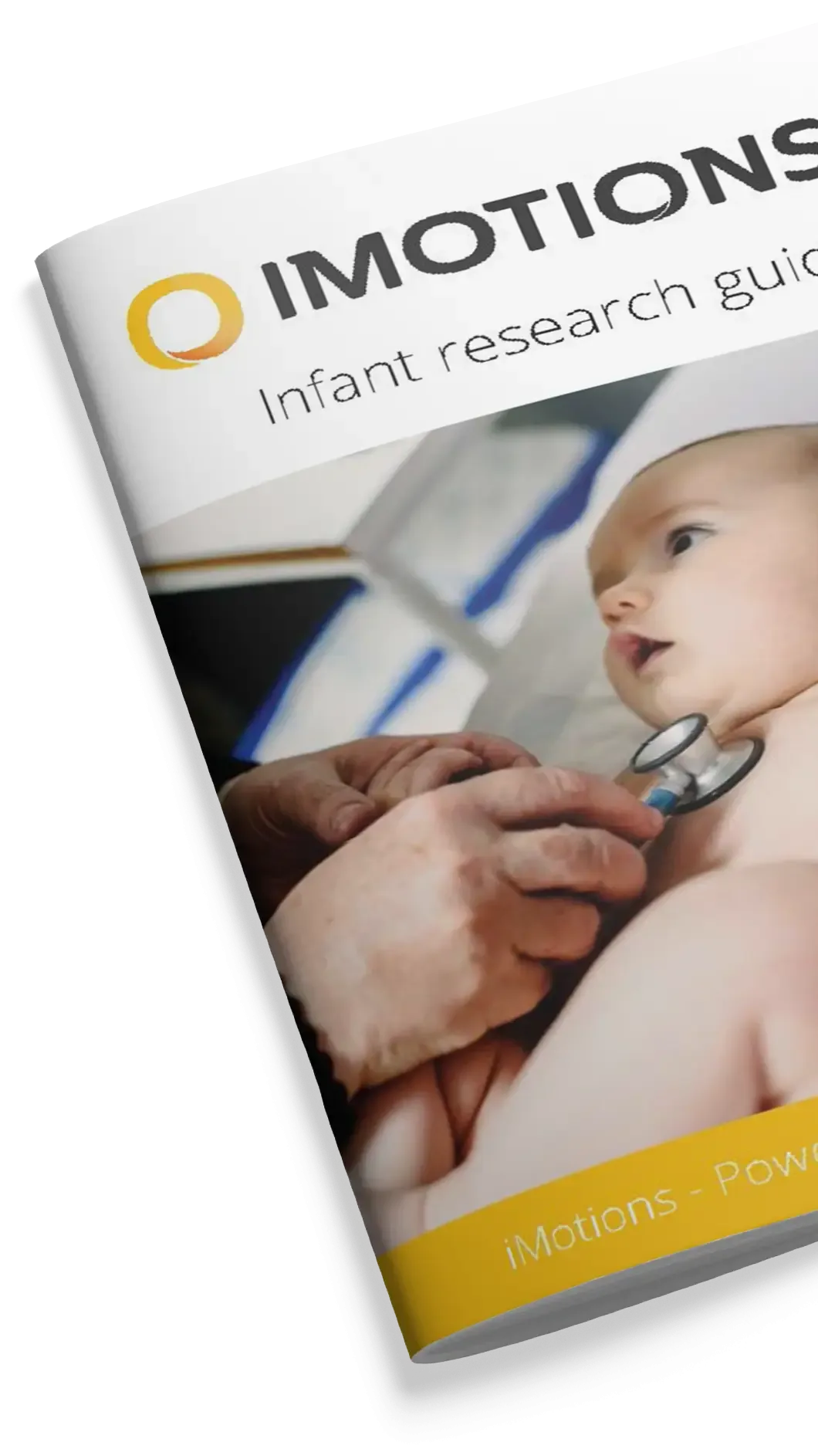
References
Adolph, K. E., & Hoch, J. E. (2019). Motor Development: Embodied, Embedded, Enculturated, and Enabling. Annual review of psychology, 70, 141–164. https://doi.org/10.1146/annurev-psych-010418-102836
Adolph, K. E., & Franchak, J. M. (2017). The development of motor behavior. Wiley interdisciplinary reviews. Cognitive science, 8(1-2), 10.1002/wcs.1430. https://doi.org/10.1002/wcs.1430
Airaksinen, M., Gallen, A., Kivi, A., Vijayakrishnan, P., Häyrinen, T., Ilén, E., Räsänen, O., Haataja, L. M., & Vanhatalo, S. (2022). Intelligent wearable allows out-of-the-lab tracking of developing motor abilities in infants. Communications medicine, 2, 69. https://doi.org/10.1038/s43856-022-00131-6
Airaksinen, M., Räsänen, O., Ilén, E., Häyrinen, T., Kivi, A., Marchi, V., Gallen, A., Blom, S., Varhe, A., Kaartinen, N., Haataja, L., & Vanhatalo, S. (2020). Automatic Posture and Movement Tracking of Infants with Wearable Movement Sensors. Scientific reports, 10(1), 169. https://doi.org/10.1038/s41598-019-56862-5
American Optometric Association (undated). Infant Vision: Birth to 24 Months of Age. https://www.aoa.org/healthy-eyes/eye-health-for-life/infant-vision?sso=y . Accessed on 6/27/24
Aslin R. N. (2012). Infant eyes: A window on cognitive development. Infancy : the official journal of the International Society on Infant Studies, 17(1), 126–140. https://doi.org/10.1111/j.1532-7078.2011.00097.x
Bae, S., Borac, S., Emre, Y., Wang, J., Wu, J., Kashyap, M., Kang, S. H., Chen, L., Moran, M., Cannon, J., Teasley, E. S., Chai, A., Liu, Y., Wadhwa, N., Krainin, M., Rubinstein, M., Maciel, A., McConnell, M. V., Patel, S., Corrado, G. S., … Po, M. J. (2022). Prospective validation of smartphone-based heart rate and respiratory rate measurement algorithms. Communications medicine, 2, 40. https://doi.org/10.1038/s43856-022-00102-x
Bánki, A., de Eccher, M., Falschlehner, L., Hoehl, S., & Markova, G. (2022). Comparing Online Webcam- and Laboratory-Based Eye-Tracking for the Assessment of Infants’ Audio-Visual Synchrony Perception. Frontiers in psychology, 12, 733933. https://doi.org/10.3389/fpsyg.2021.733933
Borjon, J. I., Abney, D. H., Yu, C., & Smith, L. B. (2021). Head and eyes: Looking behavior in 12- to 24-month-old infants. Journal of vision, 21(8), 18. https://doi.org/10.1167/jov.21.8.18
Bosquet Enlow, M., King, L., Schreier, H. M., Howard, J. M., Rosenfield, D., Ritz, T., & Wright, R. J. (2014). Maternal sensitivity and infant autonomic and endocrine stress responses. Early human development, 90(7), 377–385. https://doi.org/10.1016/j.earlhumdev.2014.04.007
Britton JW, Frey LC, Hopp JLet al., authors; St. Louis EK, Frey LC, editors. Electroencephalography (EEG): An Introductory Text and Atlas of Normal and Abnormal Findings in Adults, Children, and Infants [Internet]. Chicago: American Epilepsy Society; 2016. The Developmental EEG: Premature, Neonatal, Infant, and Children. Available from: https://www.ncbi.nlm.nih.gov/books/NBK390356/
Buthmann, J., Finik, J., & Nomura, Y. (2018). Sex differences in the relations between infant temperament and electrodermal responses in early childhood. International journal of behavioral development, 42(6), 535–542. https://doi.org/10.1177/0165025418757705
Carbone, T., Marrero, L. C., Weiss, J., Hiatt, M., & Hegyi, T. (1999). Heart rate and oxygen saturation correlates of infant apnea. Journal of perinatology : official journal of the California Perinatal Association, 19(1), 44–47. https://doi.org/10.1038/sj.jp.7200072
Center for Disease Control & Prevention (2024, May 16). Positive Parenting Tips: Infants (0-1 years). https://www.cdc.gov/child-development/positive-parenting-tips/infants.html?CDC_AAref_Val=https://www.cdc.gov/ncbddd/childdevelopment/positiveparenting/infants.html Accessed on 6/27/24.
Center for Disease Control & Prevention (2024, May 8). CDC’s Developmental Milestones. https://www.cdc.gov/ncbddd/actearly/milestones/index.html . Accessed on 6/27/24.
Centers for Disease Control & Prevention (2024, January 25). Data and Statistics on Autism Spectrum Disorder. https://www.cdc.gov/autism/data-research/?CDC_AAref_Val=https://www.cdc.gov/ncbddd/autism/data.html . Accessed on 7/11/24.
Centers for Disease Control & Prevention (2024, May 15). High Blood Pressure Facts. https://www.cdc.gov/high-blood-pressure/data-research/facts-stats/index.html#:~:text=Normal,3 . Accessed on 7/11/24.
Chen, H., Wang, Z., Lu, C., Shu, F., Chen, C., Wang, L., & Chen, W. (2023). Neonatal Seizure Detection Using a Wearable Multi-Sensor System. Bioengineering (Basel, Switzerland), 10(6), 658. https://doi.org/10.3390/bioengineering10060658
Chen, H., Xue, M., Mei, Z., Bambang Oetomo, S., & Chen, W. (2016). A Review of Wearable Sensor Systems for Monitoring Body Movements of Neonates. Sensors (Basel, Switzerland), 16(12), 2134. https://doi.org/10.3390/s16122134
Chiera, M., Cerritelli, F., Casini, A., Barsotti, N., Boschiero, D., Cavigioli, F., Corti, C. G., & Manzotti, A. (2020). Heart Rate Variability in the Perinatal Period: A Critical and Conceptual Review. Frontiers in neuroscience, 14, 561186. https://doi.org/10.3389/fnins.2020.561186
Chung, H. W., Chang, C. K., Huang, T. H., Chen, L. C., Chen, H. L., Yang, S. T., Chen, C. C., & Wang, K. (2023). Mobile Device-Based Video Screening for Infant Head Lag: An Exploratory Study. Children (Basel, Switzerland), 10(7), 1239. https://doi.org/10.3390/children10071239
Chung, H. U., Kim, B. H., Lee, J. Y., Lee, J., Xie, Z., Ibler, E. M., Lee, K., Banks, A., Jeong, J. Y., Kim, J., Ogle, C., Grande, D., Yu, Y., Jang, H., Assem, P., Ryu, D., Kwak, J. W., Namkoong, M., Park, J. B., Lee, Y., … Rogers, J. A. (2019). Binodal, wireless epidermal electronic systems with in-sensor analytics for neonatal intensive care. Science (New York, N.Y.), 363(6430), eaau0780. https://doi.org/10.1126/science.aau0780
Chung, H. U., Rwei, A. Y., Hourlier-Fargette, A., Xu, S., Lee, K., Dunne, E. C., Xie, Z., Liu, C., Carlini, A., Kim, D. H., Ryu, D., Kulikova, E., Cao, J., Odland, I. C., Fields, K. B., Hopkins, B., Banks, A., Ogle, C., Grande, D., Park, J. B., … Rogers, J. A. (2020). Skin-interfaced biosensors for advanced wireless physiological monitoring in neonatal and pediatric intensive-care units. Nature medicine, 26(3), 418–429. https://doi.org/10.1038/s41591-020-0792-9
Claiborne, A., Williams, A., Jolly, C., Isler, C., Newton, E., May, L., & George, S. (2023). Methods for analyzing infant heart rate variability: A preliminary study. Birth defects research, 115(10), 998–1006. https://doi.org/10.1002/bdr2.2177
Corbetta, D., Guan, Y., & Williams, J. L. (2012). Infant Eye-tracking in the Context of Goal-Directed Actions. Infancy : the official journal of the International Society on Infant Studies, 17(1), 102–125. https://doi.org/10.1111/j.1532-7078.2011.0093.x
Crespo-Llado, M. M., Vanderwert, R., Roberti, E., & Geangu, E. (2018). Eight-month-old infants’ behavioral responses to peers’ emotions as related to the asymmetric frontal cortex activity. Scientific reports, 8(1), 17152. https://doi.org/10.1038/s41598-018-35219-4
Cobos-Torres, J. C., Abderrahim, M., & Martínez-Orgado, J. (2018). Non-Contact, Simple Neonatal Monitoring by Photoplethysmography. Sensors (Basel, Switzerland), 18(12), 4362. https://doi.org/10.3390/s18124362
Dalton, K. M., Nacewicz, B. M., Johnstone, T., Schaefer, H. S., Gernsbacher, M. A., Goldsmith, H. H., Alexander, A. L., & Davidson, R. J. (2005). Gaze fixation and the neural circuitry of face processing in autism. Nature neuroscience, 8(4), 519–526. https://doi.org/10.1038/nn1421
Dzwilewski, K. L. C., Merced-Nieves, F. M., Aguiar, A., Korrick, S. A., & Schantz, S. L. (2020). Characterization of performance on an automated visual recognition memory task in 7.5-month-old infants. Neurotoxicology and teratology, 81, 106904. https://doi.org/10.1016/j.ntt.2020.106904
Endevelt-Shapira, Y., & Feldman, R. (2023). Mother-Infant Brain-to-Brain Synchrony Patterns Reflect Caregiving Profiles. Biology, 12(2), 284. https://doi.org/10.3390/biology12020284
Eriksson, M., Storm, H., Fremming, A., & Schollin, J. (2008). Skin conductance compared to a combined behavioural and physiological pain measure in newborn infants. Acta paediatrica (Oslo, Norway : 1992), 97(1), 27–30. https://doi.org/10.1111/j.1651-2227.2007.00586.x
Onal Ertugrul, I., Ahn, Y. A., Bilalpur, M., Messinger, D. S., Speltz, M. L., & Cohn, J. F. (2023). Infant AFAR: Automated facial action recognition in infants. Behavior research methods, 55(3), 1024–1035. https://doi.org/10.3758/s13428-022-01863-y
Govindaswami, B., Jegatheesan, P., Song, D. (2012). Oxygen Saturation Screening for Critical Congenital Heart Disease. Neoreviews 13 (12): e724–e731. https://doi.org/10.1542/neo.13-12-e724
Gervain, J., Minagawa, Y., Emberson, L., & Lloyd-Fox, S. (2023). Using functional near-infrared spectroscopy to study the early developing brain: future directions and new challenges. Neurophotonics, 10(2), 023519. https://doi.org/10.1117/1.NPh.10.2.023519
Gredebäck, G., Johnson, S., & von Hofsten, C. (2010). Eye tracking in infancy research. Developmental neuropsychology, 35(1), 1–19. https://doi.org/10.1080/87565640903325758
Fairchild, K. D., & O’Shea, T. M. (2010). Heart rate characteristics: physiomarkers for detection of late-onset neonatal sepsis. Clinics in perinatology, 37(3), 581–598. https://doi.org/10.1016/j.clp.2010.06.002
Filippa, M., Benis, D., Adam-Darque, A., Grandjean, D., & Hüppi, P. S. (2023). Preterm infants show an atypical processing of the mother’s voice. Brain and cognition, 173, 106104. https://doi.org/10.1016/j.bandc.2023.106104
Forestell, C. A., & Mennella, J. A. (2012). More than just a pretty face. The relationship between infant’s temperament, food acceptance, and mothers’ perceptions of their enjoyment of food. Appetite, 58(3), 1136–1142. https://doi.org/10.1016/j.appet.2012.03.005
Ham, J., & Tronick, E. (2008). A procedure for the measurement of infant skin conductance and its initial validation using clap induced startle. Developmental psychobiology, 50(6), 626–631. https://doi.org/10.1002/dev.20317
Harper, R. M., Hoppenbrouwers, T., Sterman, M. B., McGinty, D. J., & Hodgman, J. (1976). Polygraphic studies of normal infants during the first six months of life. I. Heart rate and variability as a function of state. Pediatric research, 10(11), 945–948. https://doi.org/10.1203/00006450-197611000-00008
Healthline (2022). What are Normal Respiratory Rates for Adults and Children? https://www.healthline.com/health/normal-respiratory-rate . Accessed on 7/11/24.
Healthline (2024). What is the Normal Body Temperature Range? https://www.healthline.com/health/what-is-normal-body-temperature . Accessed on 7/11/24.
Islam, B., McElwain, N. L., Li, J., Davila, M. I., Hu, Y., Hu, K., Bodway, J. M., Dhekne, A., Roy Choudhury, R., & Hasegawa-Johnson, M. (2024). Preliminary Technical Validation of LittleBeats™: A Multimodal Sensing Platform to Capture Cardiac Physiology, Motion, and Vocalizations. Sensors (Basel, Switzerland), 24(3), 901. https://doi.org/10.3390/s24030901
Izard, C. E., Fantauzzo, C. A., Castle, J. M., Haynes, O. M., Rayias, M. F., & Putnam, P. H. (1995). The ontogeny and significance of infants’ facial expressions in the first 9 months of life. Developmental Psychology, 31(6), 997–1013. https://doi.org/10.1037/0012-1649.31.6.997
Ji, C., Mudiyanselage, T.B., Gao, Y. et al. A review of infant cry analysis and classification. J AUDIO SPEECH MUSIC PROC. 2021, 8 (2021). https://doi.org/10.1186/s13636-021-00197-5
Jones, E. J. H., Goodwin, A., Orekhova, E., Charman, T., Dawson, G., Webb, S. J., & Johnson, M. H. (2020). Infant EEG theta modulation predicts childhood intelligence. Scientific reports, 10(1), 11232. https://doi.org/10.1038/s41598-020-67687-y
Joshi, R., Kommers, D., Oosterwijk, L., Feijs, L., van Pul, C., & Andriessen, P. (2020). Predicting Neonatal Sepsis Using Features of Heart Rate Variability, Respiratory Characteristics, and ECG-Derived Estimates of Infant Motion. IEEE journal of biomedical and health informatics, 24(3), 681–692. https://doi.org/10.1109/JBHI.2019.2927463
Kaul, Y. F., Rosander, K., von Hofsten, C., Brodd, K. S., Holmström, G., Kaul, A., Böhm, B., & Hellström-Westas, L. (2016). Visual tracking in very preterm infants at 4 mo predicts neurodevelopment at 3 y of age. Pediatric research, 80(1), 35–42. https://doi.org/10.1038/pr.2016.37
Killinc, O., & Snyder, C.S. (2020, February 14). Fast, Slow, and Irregular Heartbeats. HealthyChildren.org. https://www.healthychildren.org/English/health-issues/conditions/heart/Pages/Irregular-Heartbeat-Arrhythmia.aspx . Accessed on 7/11/24.
Kim, J., Yoo, S., Liu, C. et al. Skin-interfaced wireless biosensors for perinatal and paediatric health. Nat Rev Bioeng 1, 631–647 (2023). https://doi.org/10.1038/s44222-023-00090-0
King, L. (2024). What is Heart Rate Variability? WebMD. https://www.webmd.com/heart/what-is-heart-rate-variability Accesed on 7/11/24.
Kleiger, R. E., Stein, P. K., & Bigger, J. T., Jr (2005). Heart rate variability: measurement and clinical utility. Annals of noninvasive electrocardiology : the official journal of the International Society for Holter and Noninvasive Electrocardiology, Inc, 10(1), 88–101. https://doi.org/10.1111/j.1542-474X.2005.10101.x
Klin, A., Jones, W., Schultz, R., Volkmar, F., & Cohen, D. (2002). Visual fixation patterns during viewing of naturalistic social situations as predictors of social competence in individuals with autism. Archives of general psychiatry, 59(9), 809–816. https://doi.org/10.1001/archpsyc.59.9.809
Kuhl, P. K., & Meltzoff, A. N. (1996). Infant vocalizations in response to speech: vocal imitation and developmental change. The Journal of the Acoustical Society of America, 100(4 Pt 1), 2425–2438. https://doi.org/10.1121/1.417951
Kulvicius, T., Zhang, D., Nielsen-Saines, K., Bölte, S., Kraft, M., Einspieler, C., Poustka, L., Wörgötter, F., & Marschik, P. B. (2023). Infant movement classification through pressure distribution analysis. Communications medicine, 3(1), 112. https://doi.org/10.1038/s43856-023-00342-5
Kumar, N., Akangire, G., Sullivan, B., Fairchild, K., & Sampath, V. (2020). Continuous vital sign analysis for predicting and preventing neonatal diseases in the twenty-first century: big data to the forefront. Pediatric research, 87(2), 210–220. https://doi.org/10.1038/s41390-019-0527-0
Latremouille, S., Lam, J., Shalish, W., & Sant’Anna, G. (2021). Neonatal heart rate variability: a contemporary scoping review of analysis methods and clinical applications. BMJ open, 11(12), e055209. https://doi.org/10.1136/bmjopen-2021-055209
Lee, A.R. (2024). What is a Normal Heart Rate Variability? Health. https://www.health.com/condition/heart-disease/heart-rate-variability#:~:text=The%20average%20%22good%2C%22%20or,than%20115%2C000%20times%20a%20day. Accessed on 7/11/24.
Leppänen, J. M., Butcher, J. W., Godbout, C., Stephenson, K., Hendrixson, D. T., Griswold, S., Rogers, B. L., Webb, P., Koroma, A. S., & Manary, M. J. (2022). Assessing infant cognition in field settings using eye-tracking: a pilot cohort trial in Sierra Leone. BMJ open, 12(2), e049783. https://doi.org/10.1136/bmjopen-2021-049783
Levine, H. (2023, February 2). What Your Heart Rate Says About Your Health. AARP. https://www.aarp.org/health/healthy-living/info-2023/what-is-a-healthy-heart-rate.html?cmp=KNC-DSO-COR-Health-HeartHealth-NonBrand-Phrase-44175-Bing-HEALTH-Healthy-Heart-Rate-Phrase-NonBrand&&msclkid=c6c433cd09a717a4488d5542d26b9d56&utm_source=bing&utm_medium=cpc&utm_campaign=Health-HeartHealth-NonBrand-Phrase&utm_term=bpm%20heart%20rate&utm_content=Healthy-Heart-Rate&gclid=c6c433cd09a717a4488d5542d26b9d56&gclsrc=3p.ds . Accessed on 7/11/24.
Li, J., Hasegawa-Johnson, M., & McElwain, N. L. (2021). Analysis of acoustic and voice quality features for the classification of infant and mother vocalizations. Speech communication, 133, 41–61. https://doi.org/10.1016/j.specom.2021.07.010
Liu, X., Fromm, J., Patel, S., McDuf, D. (2020). Multi-task temporal shift attention networks for on-device contactless vitals measurement. Advances in Neural Information Processing Systems, 33:19400-19411.
Lloyd-Fox, S., Papademetriou, M., Darboe, M. K., Everdell, N. L., Wegmuller, R., Prentice, A. M., Moore, S. E., & Elwell, C. E. (2014). Functional near infrared spectroscopy (fNIRS) to assess cognitive function in infants in rural Africa. Scientific reports, 4, 4740. https://doi.org/10.1038/srep04740
Löscher, W. N., Einspieler, C., Klug, E. M., Haidmayer, R., Gallasch, E., Kurz, R., & Kenner, T. (1990). Neurological status, sleep apnea frequency and blood oxygenation in six weeks old infants. Early human development, 24(2), 119–130. https://doi.org/10.1016/0378-3782(90)90142-6
Malik F, Marwaha R. Cognitive Development. [Updated 2023 Apr 23]. In: StatPearls [Internet]. Treasure Island (FL): StatPearls Publishing; 2024 Jan-. Available from: https://www.ncbi.nlm.nih.gov/books/NBK537095/
Maenner, M. J., Warren, Z., Williams, A. R., Amoakohene, E., Bakian, A. V., Bilder, D. A., Durkin, M. S., Fitzgerald, R. T., Furnier, S. M., Hughes, M. M., Ladd-Acosta, C. M., McArthur, D., Pas, E. T., Salinas, A., Vehorn, A., Williams, S., Esler, A., Grzybowski, A., Hall-Lande, J., Nguyen, R. H. N., … Shaw, K. A. (2023). Prevalence and Characteristics of Autism Spectrum Disorder Among Children Aged 8 Years – Autism and Developmental Disabilities Monitoring Network, 11 Sites, United States, 2020. Morbidity and mortality weekly report. Surveillance summaries (Washington, D.C. : 2002), 72(2), 1–14. https://doi.org/10.15585/mmwr.ss7202a1
Manning, J., Thakur, D., Bowler, K., Inamdar, K., Chu, V.W. (2023). Validation of Parent Recall & Accelerometer Measure for Tracking Tummy Time at Home in Term & Preterm Infants. Am J Occup Ther Vol. 77(Supplement_2), 7711500016p1. doi: https://doi.org/10.5014/ajot.2023.77S2-PO16
Mehta, S. K., Super, D. M., Connuck, D., Salvator, A., Singer, L., Fradley, L. G., Harcar-Sevcik, R. A., Kirchner, H. L., & Kaufman, E. S. (2002). Heart rate variability in healthy newborn infants. The American journal of cardiology, 89(1), 50–53. https://doi.org/10.1016/s0002-9149(01)02162-2
Memon, S.F., Memon, M. and Bhatti, S. (2020), Wearable technology for infant health monitoring: a survey. IET Circuits Devices Syst., 14: 115-129. https://doi.org/10.1049/iet-cds.2018.5447
Mount Sinai (undated). High Blood Pressure – Infants. https://www.mountsinai.org/health-library/diseases-conditions/high-blood-pressure-infants#:~:text=Exposure%20to%20illegal%20drugs%20such,Rapid%20breathing. Accessed on 7/11/24.
Mueller, I., Snidman, N., DiCorcia, J. A., & Tronick, E. (2021). Acute Maternal Stress Disrupts Infant Regulation of the Autonomic Nervous System and Behavior: A CASP Study. Frontiers in psychiatry, 12, 714664. https://doi.org/10.3389/fpsyt.2021.714664
National Institute of Child Health and Human Development (2021, April 19). Early Intervention for Autism. https://www.nichd.nih.gov/health/topics/autism/conditioninfo/treatments/early-intervention . Accessed on 7/11/24.
Nayak CS, Anilkumar AC. Neonatal EEG. [Updated 2024 Feb 17]. In: StatPearls [Internet]. Treasure Island (FL): StatPearls Publishing; 2024 Jan-. Available from: https://www.ncbi.nlm.nih.gov/books/NBK536953/
Ness, S. L., Manyakov, N. V., Bangerter, A., Lewin, D., Jagannatha, S., Boice, M., Skalkin, A., Dawson, G., Janvier, Y. M., Goodwin, M. S., Hendren, R., Leventhal, B., Shic, F., Cioccia, W., & Pandina, G. (2017). JAKE® Multimodal Data Capture System: Insights from an Observational Study of Autism Spectrum Disorder. Frontiers in neuroscience, 11, 517. https://doi.org/10.3389/fnins.2017.00517
Oliveira, V., von Rosenberg, W., Montaldo, P., Adjei, T., Mendoza, J., Shivamurthappa, V., Mandic, D., & Thayyil, S. (2019). Early Postnatal Heart Rate Variability in Healthy Newborn Infants. Frontiers in physiology, 10, 922. https://doi.org/10.3389/fphys.2019.00922
Perochon, S., Di Martino, J. M., Carpenter, K. L. H., Compton, S., Davis, N., Eichner, B., Espinosa, S., Franz, L., Krishnappa Babu, P. R., Sapiro, G., & Dawson, G. (2023). Early detection of autism using digital behavioral phenotyping. Nature medicine, 29(10), 2489–2497. https://doi.org/10.1038/s41591-023-02574-3
Pitchford, E. A., Ketcheson, L. R., Kwon, H. J., & Ulrich, D. A. (2017). Minimum Accelerometer Wear Time in Infants: A Generalizability Study. Journal of physical activity & health, 14(6), 421–428. https://doi.org/10.1123/jpah.2016-0395
Rash, J. A., Thomas, J. C., Campbell, T. S., Letourneau, N., Granger, D. A., Giesbrecht, G. F., & APrON Study Team (2016). Developmental origins of infant stress reactivity profiles: A multi-system approach. Developmental psychobiology, 58(5), 578–599. https://doi.org/10.1002/dev.21403
Rayson, H., Bonaiuto, J. J., Ferrari, P. F., & Murray, L. (2017). Early maternal mirroring predicts infant motor system activation during facial expression observation. Scientific reports, 7(1), 11738. https://doi.org/10.1038/s41598-017-12097-w
Reuter, S., Moser, C., & Baack, M. (2014). Respiratory distress in the newborn. Pediatrics in review, 35(10), 417–429. https://doi.org/10.1542/pir.35-10-417
Ricardo, L. I. C., DA Silva, I. C. M., Martins, R. C., Wendt, A., Gonçalves, H., Hallal, P. R. C., & Wehrmeister, F. C. (2018). Protocol for Objective Measurement of Infants’ Physical Activity using Accelerometry. Medicine and science in sports and exercise, 50(5), 1084–1092. https://doi.org/10.1249/MSS.0000000000001512
Richard, C. A., & Mosko, S. S. (2004). Mother-infant bedsharing is associated with an increase in infant heart rate. Sleep, 27(3), 507–511. https://doi.org/10.1093/sleep/27.3.507
Ritz, T., Bosquet Enlow, M., Schulz, S. M., Kitts, R., Staudenmayer, J., & Wright, R. J. (2012). Respiratory sinus arrhythmia as an index of vagal activity during stress in infants: respiratory influences and their control. PloS one, 7(12), e52729. https://doi.org/10.1371/journal.pone.0052729
Sahni, R., Gupta, A., Ohira-Kist, K., & Rosen, T. S. (2003). Motion resistant pulse oximetry in neonates. Archives of disease in childhood. Fetal and neonatal edition, 88(6), F505–F508. https://doi.org/10.1136/fn.88.6.f505
Schechtman, V. L., Harper, R. M., Kluge, K. A., Wilson, A. J., Hoffman, H. J., & Southall, D. P. (1988). Cardiac and respiratory patterns in normal infants and victims of the sudden infant death syndrome. Sleep, 11(5), 413–424. https://doi.org/10.1093/sleep/11.5.413
Sloan, A. T., Jones, N. A., & Kelso, J. A. S. (2023). Meaning from movement and stillness: Signatures of coordination dynamics reveal infant agency. Proceedings of the National Academy of Sciences of the United States of America, 120(39), e2306732120. https://doi.org/10.1073/pnas.2306732120
Stanford Medicine Children Health (undated). Age Appropriate Speech and Hearing Milestones. https://www.stanfordchildrens.org/en/topic/default?id=age-appropriate-speech-and-hearing-milestones-90-P02169 . Accessed on 6/27/24.
Storm, H. Electrodermal activity measures the pain response from heel lance in premature infants from 29 weeks gestational age. Pediatr Res 45, 899 (1999). https://doi.org/10.1203/00006450-199906000-00094
Tamura T, Maeda Y, Sekine M, Yoshida M. Wearable Photoplethysmographic Sensors—Past and Present. Electronics. 2014; 3(2):282-302. https://doi.org/10.3390/electronics3020282
Thorup, E., Nyström, P., Gredebäck, G., Bölte, S., Falck-Ytter, T., & EASE Team (2016). Altered gaze following during live interaction in infants at risk for autism: an eye tracking study. Molecular autism, 7, 12. https://doi.org/10.1186/s13229-016-0069-9
Valentine, S., Cunningham, A. C., Klasmer, B., Dabbah, M., Balabanovic, M., Aral, M., Vahdat, D., & Plans, D. (2022). Smartphone movement sensors for the remote monitoring of respiratory rates: Technical validation. Digital health, 8, 20552076221089090. https://doi.org/10.1177/20552076221089090
Visnovcova, Z., Kozar, M., Kuderava, Z., Zibolen, M., Ferencova, N., & Tonhajzerova, I. (2022). Entropy Analysis of Neonatal Electrodermal Activity during the First Three Days after Birth. Entropy (Basel, Switzerland), 24(3), 422. https://doi.org/10.3390/e24030422
Weiss, S. J., Keeton, V. F., Leung, C., & Niemann, S. (2024). Infant emotion regulation in the context of stress: Effects of heart rate variability and temperament. Stress and health : journal of the International Society for the Investigation of Stress, e3373. Advance online publication. https://doi.org/10.1002/smi.3373
Weibley, H., Di Filippo, M., Liu, X., Lazenby, L., Goscha, J., Ferreira, A., Muscalu, L., & Rader, N. (2021). fNIRS Monitoring of Infant Prefrontal Cortex During Crawling and an Executive Functioning Task. Frontiers in behavioral neuroscience, 15, 675366. https://doi.org/10.3389/fnbeh.2021.675366
Wen, T. H., Cheng, A., Andreason, C., Zahiri, J., Xiao, Y., Xu, R., Bao, B., Courchesne, E., Barnes, C. C., Arias, S. J., & Pierce, K. (2022). Large scale validation of an early-age eye-tracking biomarker of an autism spectrum disorder subtype. Scientific reports, 12(1), 4253. https://doi.org/10.1038/s41598-022-08102-6
Wikipedia (2024, June 23). Infant. https://en.wikipedia.org/wiki/Infant . Accessed on 6/27/24.
Xu, S., Rwei, A. Y., Vwalika, B., Chisembele, M. P., Stringer, J. S. A., Ginsburg, A. S., & Rogers, J. A. (2021). Wireless skin sensors for physiological monitoring of infants in low-income and middle-income countries. The Lancet. Digital health, 3(4), e266–e273. https://doi.org/10.1016/S2589-7500(21)00001-7
Yamamoto, H., Sato, A., & Itakura, S. (2019). Eye tracking in an everyday environment reveals the interpersonal distance that affords infant-parent gaze communication. Scientific reports, 9(1), 10352. https://doi.org/10.1038/s41598-019-46650-6
Yasin, S., Draz, U., Ali, T., Shahid, K., Abid, A. et al. (2022). Automated speech recognition system to detect babies’ feelings through feature analysis. Computers, Materials & Continua, 73(2), 4349-4367. https://doi.org/10.32604/cmc.2022.028251
Zaharieva, M. S., Salvadori, E. A., Messinger, D. S., Visser, I., & Colonnesi, C. (2024). Automated facial expression measurement in a longitudinal sample of 4- and 8-month-olds: Baby FaceReader 9 and manual coding of affective expressions. Behavior research methods, 10.3758/s13428-023-02301-3. Advance online publication. https://doi.org/10.3758/s13428-023-02301-3
Zhao, F., Li, M., Jiang, Z., Tsien, J. Z., & Lu, Z. (2016). Camera-Based, Non-Contact, Vital-Signs Monitoring Technology May Provide a Way for the Early Prevention of SIDS in Infants. Frontiers in neurology, 7, 236. https://doi.org/10.3389/fneur.2016.00236
Zhao, F., Li, M., Qian, Y., & Tsien, J. Z. (2013). Remote measurements of heart and respiration rates for telemedicine. PloS one, 8(10), e71384. https://doi.org/10.1371/journal.pone.0071384
Zhou, L., Guess, M., Kim, K. R., & Yeo, W. H. (2024). Skin-interfacing wearable biosensors for smart health monitoring of infants and neonates. Communications materials, 5(1), 72. https://doi.org/10.1038/s43246-024-00511-6
Zhu, Z., Liu, T., Li, G., Li, T., & Inoue, Y. (2015). Wearable sensor systems for infants. Sensors (Basel, Switzerland), 15(2), 3721–3749. https://doi.org/10.3390/s150203721
Zuzarte, I., Indic, P., Sternad, D., & Paydarfar, D. (2019). Quantifying Movement in Preterm Infants Using Photoplethysmography. Annals of biomedical engineering, 47(2), 646–658. https://doi.org/10.1007/s10439-018-02135-7