Table of Contents
- Introduction
- Chapter 1: Core Concepts in Human Factors Ergonomics
- Chapter 2: The Role of Ergonomics in Human Behavior Research
- Chapter 3: Biosensors in Human Factors Research
- Chapter 4: Software Solutions for Human Factors Ergonomics
- Chapter 5: Applications of Human Factors Ergonomics in Different Industries
- Chapter 6: Challenges and Future Directions in Human Factors Ergonomics
- Appendix
iMotions Ergonomics Research Lab
Synchronize data collection from multiple biosensors, allowing researchers to investigate complex research questions in innovative and time saving ways.
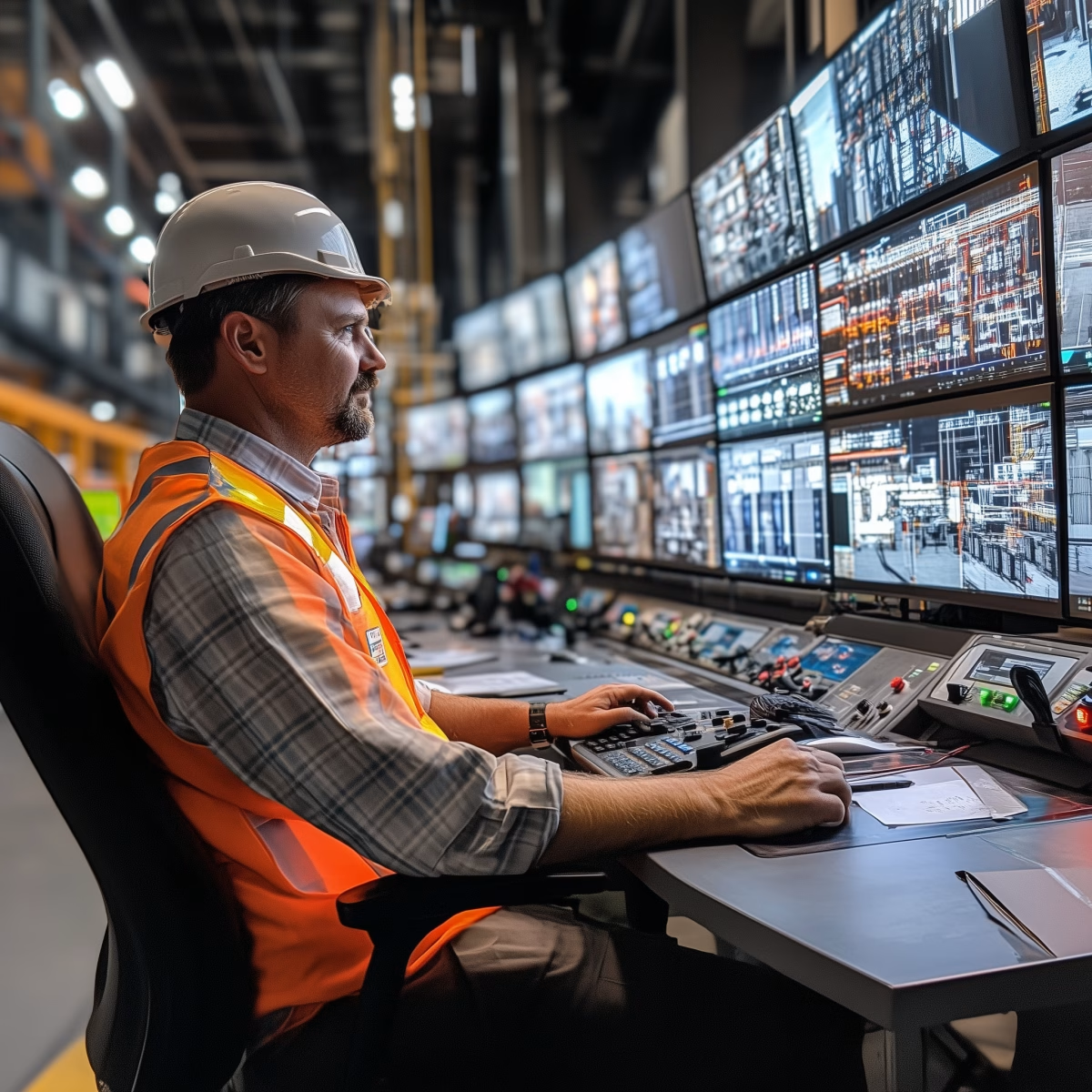
Introduction
Human Factors Ergonomics (HFE) is a multidisciplinary field focused on optimizing the design of systems, environments, and products to fit human capabilities and limitations. By integrating principles from psychology, engineering, physiology, and design, HFE seeks to enhance safety, performance, efficiency, and well-being in a wide range of contexts. Whether designing workstations that reduce physical strain, developing user-friendly software interfaces, or improving the layout of high-stress environments like hospitals or cockpits, HFE aims to create systems that align with how people think, feel, and behave.
At its core, ergonomics revolves around the concept of “fit”—the notion that tools, environments, and processes should be designed to accommodate human needs, not the other way around. This fit applies across physical, cognitive, and sensory domains, acknowledging that people have unique abilities and constraints that must be considered during the design process. Poor ergonomic design can lead to physical injuries, cognitive overload, emotional stress, and decreased productivity. Conversely, well-designed systems can improve comfort, reduce errors, and enhance overall performance.
In recent years, the field has been transformed by the advent of new technologies, particularly the use of biosensors and software platforms that provide objective insights into human behavior. Biosensors, such as eye trackers, electroencephalography (EEG) devices, electrodermal activity (EDA) monitors, and facial expression analysis tools, offer real-time data on users’ physiological, cognitive, and emotional states. By integrating this data with advanced software solutions, like those offered by iMotions A/S, ergonomics researchers can gain a deeper understanding of how individuals interact with systems and environments, leading to more effective and tailored design solutions.
The purpose of this guide is to provide a comprehensive overview of Human Factors Ergonomics, covering the foundational principles of the field and exploring how modern technology is shaping its future. The guide will examine core concepts of ergonomics, delve into the role of HFE in human behavior research, and highlight how biosensors and software platforms are driving innovation in this area. Furthermore, it will explore the practical applications of HFE across different industries, from healthcare and transportation to workplace design and consumer products, illustrating the broad impact of ergonomics on everyday life.
As we advance into a future driven by complex systems, artificial intelligence, and immersive technologies like virtual reality (VR), the need for ergonomically sound design is more critical than ever. The challenges and opportunities presented by these innovations will shape the direction of HFE, making it an essential field for ensuring that human well-being remains at the center of technological progress.
This guide will serve as both a reference and a roadmap, outlining the current state of Human Factors Ergonomics while offering insights into its future directions. Whether you are a researcher, designer, engineer, or practitioner, this guide aims to equip you with the knowledge needed to apply ergonomic principles effectively in your work, ultimately improving safety, performance, and user satisfaction across diverse domains.
Chapter 1: Core Concepts in Human Factors Ergonomics
1.1 Human Capabilities and Limitations
At the core of Human Factors Ergonomics (HFE) lies the understanding of human capabilities and limitations. Effective ergonomics involves designing systems, environments, and products that align with the physical, cognitive, and sensory capacities of individuals, ensuring that interactions are safe, efficient, and comfortable. This section provides a comprehensive overview of these aspects and their implications for design.
1.1.1 Physical Ergonomics
Physical ergonomics focuses on the human body’s capabilities and its interactions with the physical environment. This branch of ergonomics is concerned with factors such as human anatomy, anthropometry (body measurements), biomechanics, and the physical demands placed on the human body in various settings, from office environments to industrial workspaces.
- Human Dimensions and Anthropometry: To create effective ergonomic designs, it is essential to understand the variation in human body dimensions. Anthropometric data allows designers to tailor workspaces, tools, and products to fit the target population. This prevents discomfort, fatigue, and long-term musculoskeletal issues caused by poor fit or reachability.
- Example: An office chair designed based on average body dimensions allows for proper posture, reducing strain on the back, neck, and shoulders.
- Strength and Mobility: Understanding human strength capacities and ranges of motion is critical for designing tools and tasks that do not exceed physical limitations. Designs should minimize the risk of repetitive strain injuries, overexertion, or awkward postures.
- Example: Lifting aids in industrial environments are designed to accommodate typical human strength limits to reduce the risk of injuries.
1.1.2 Cognitive Ergonomics
Cognitive ergonomics is concerned with how mental processes, such as perception, memory, reasoning, and motor response, affect interactions with systems and environments. This field is critical for the design of user interfaces, control systems, and any environment where mental workload and decision-making are central to task performance.
- Mental Workload: Cognitive workload refers to the mental effort required to complete a task. Overloading the human cognitive system can lead to errors, decreased performance, and stress. Ergonomic design aims to optimize task complexity and interface design to align with cognitive limitations.
- Example: In aviation, cockpit designs are simplified to ensure that pilots can manage essential information without being overloaded by excessive stimuli.
- Attention and Vigilance: Human attention is limited in both scope and duration. Designing systems that help maintain focus and prevent lapses in attention is crucial, especially in high-risk environments like healthcare or transportation.
- Example: In driving, in-vehicle displays are designed to provide critical information while minimizing distractions to ensure the driver’s focus remains on the road.
- Memory and Decision-Making: Human memory has limitations in terms of capacity and retention. Systems should be designed to reduce reliance on memory through automation, prompts, or clear labeling to enhance decision-making.
- Example: A well-designed software interface includes shortcuts, visual cues, and menus to reduce the cognitive load on users, allowing them to navigate complex systems more efficiently.
1.1.3 Sensory Ergonomics
Sensory ergonomics deals with how sensory systems—such as vision, hearing, and touch—interact with the environment. Sensory input is critical in how humans perceive and respond to their surroundings, and ergonomic designs should consider sensory limitations to improve user experience and prevent sensory overload.
- Visual Ergonomics: Vision is the dominant sense in most human activities, and visual ergonomics focuses on optimizing lighting, display design, and visual interfaces. Poor lighting conditions or poorly designed visual displays can lead to eye strain, fatigue, and reduced task accuracy.
- Example: Screen designs with appropriate contrast, font size, and minimal glare help reduce eye strain in digital work environments.
- Auditory Ergonomics: Sound is another critical component of sensory input. In noisy environments, it’s essential to control noise levels to avoid hearing damage and to ensure that important auditory signals are distinguishable.
- Example: In industrial settings, auditory warnings must be clearly audible even in noisy conditions, while workers are protected from excessive noise exposure through hearing protection measures.
- Tactile Ergonomics: Touch is important for control and feedback in human-system interactions, especially in manual tasks. Understanding how tactile feedback can be optimized ensures better control precision and prevents errors.
- Example: Touchscreen devices with haptic feedback allow users to confirm input actions, reducing the chance of mistakes in data entry.
1.2 Human-System Interaction
Human-System Interaction (HSI) is the foundational element of HFE. This concept examines how humans interact with systems, products, or environments, emphasizing the need to design with the user in mind to improve usability and performance while minimizing errors.
1.2.1 Usability and Intuitive Design Principles
Usability refers to how effectively, efficiently, and satisfactorily users can interact with a product or system. A well-designed interface or system is intuitive, meaning users can easily understand and operate it without requiring extensive training.
- Learnability: The ease with which new users can operate a system. Intuitive design reduces the learning curve, enabling users to become proficient with minimal instruction.
- Example: A smartphone interface designed with consistent icons and simple gestures allows users to navigate the system naturally, even without prior experience.
- Efficiency: Efficient systems allow users to achieve their goals with minimal effort and time. Ergonomically designed interfaces minimize unnecessary steps and provide direct paths to completing tasks.
- Example: In online forms, autofill features and error-prevention prompts help users complete tasks more quickly and accurately.
1.2.2 User-Centered Design (UCD)
User-centered design (UCD) is a design philosophy that places the user at the forefront of the design process. UCD involves understanding the needs, preferences, and limitations of the end-users and incorporating these insights into design decisions.
- Iterative Design Process: UCD relies on an iterative process that involves designing, testing, and refining a product based on user feedback. This ensures that the system evolves to meet user needs more effectively.
- Example: In software development, beta versions are released to small user groups for testing, and feedback is used to improve the final product.
- User Testing and Feedback: Regular user testing, combined with observational studies, helps designers identify pain points in the user experience. Ergonomics research ensures that these issues are addressed to enhance the overall usability and satisfaction of the product.
- Example: Car manufacturers use user testing to refine dashboard layouts, ensuring controls are easy to reach and intuitive to use.
1.2.3 Designing for Diverse Populations
Ergonomic design must account for human diversity, including variations in age, gender, physical ability, and cultural backgrounds. Ensuring inclusivity in design is critical to making products and systems usable by a broader range of individuals.
- Age and Physical Abilities: As populations age, ergonomics must adapt to cater to older adults who may have reduced strength, mobility, and sensory acuity. Similarly, designs must accommodate individuals with disabilities to ensure accessibility.
- Example: In workplace design, adjustable desks and chairs allow for variations in height, posture preferences, and mobility requirements.
- Cultural Differences: Cultural differences affect how people perceive and interact with systems. Ergonomic designs must consider these variations to ensure that systems are intuitive and accessible across different regions.
- Example: In product packaging, color schemes and symbols might be interpreted differently across cultures, so it is essential to tailor these designs to avoid confusion or miscommunication.
1.3 Environmental Factors
The environments in which humans operate significantly affect their performance, comfort, and safety. Human factors ergonomics pays close attention to environmental factors such as lighting, noise, and temperature, as well as the design of physical spaces.
1.3.1 Lighting
Proper lighting is essential to ensure visibility, reduce eye strain, and maintain concentration. Both natural and artificial lighting must be optimized for the tasks being performed.
- Task Lighting: Task-specific lighting provides the right illumination where it is needed, reducing the need for individuals to strain their eyes or change their posture to see properly.
- Example: Adjustable desk lamps provide focused lighting for reading or detailed work, reducing visual strain in office environments.
1.3.2 Noise
Noise is a critical environmental factor in ergonomic design. Excessive noise can lead to fatigue, reduced focus, and hearing damage, while appropriate noise control measures can enhance productivity and comfort.
- Acoustic Control: In work environments, ergonomic design focuses on controlling background noise through soundproofing, noise barriers, or quiet zones.
- Example: Open-plan offices use sound-absorbing panels to reduce ambient noise levels, allowing for more focused work without excessive distractions.
1.3.3 Temperature and Climate Control
Thermal comfort affects human performance and well-being. An ergonomically designed environment ensures that temperature and air quality are kept within optimal ranges to avoid discomfort and fatigue.
- Thermal Zoning: Different individuals may have different comfort preferences, so ergonomic designs often allow for adjustable climate control within personal workspaces.
- Example: In modern offices, personal fans or heaters are provided to allow employees to adjust their immediate environment to their comfort.
Chapter 2: The Role of Ergonomics in Human Behavior Research
Human behavior research plays a central role in understanding how individuals interact with systems, environments, and products. By leveraging ergonomic principles, researchers can identify the factors that influence performance, safety, and well-being. Human Factors Ergonomics (HFE) seeks to optimize these interactions by designing with human capabilities and limitations in mind. In this chapter, we will explore how ergonomics informs human behavior research, with a focus on human error, performance optimization, and the critical tools used in these studies.
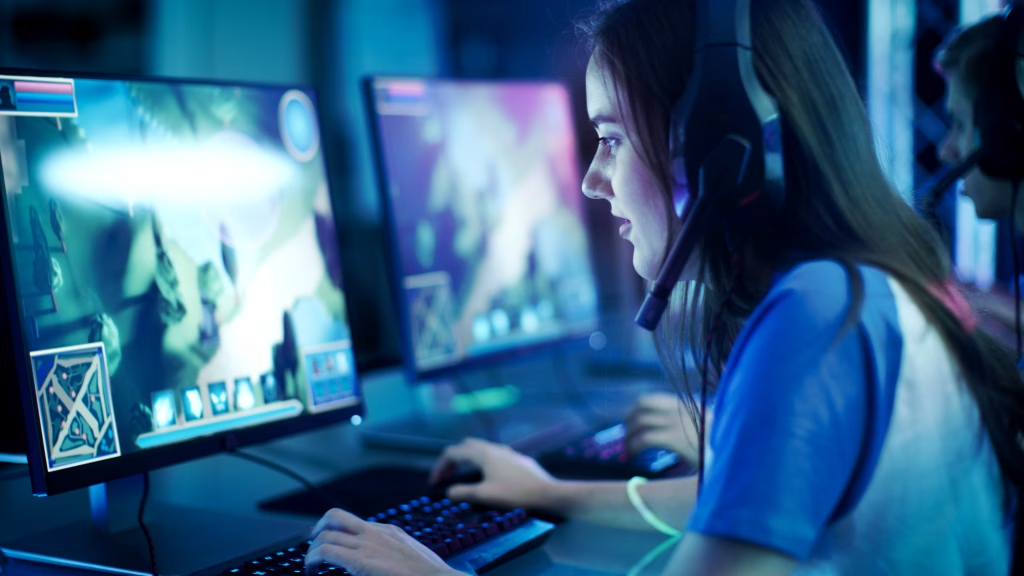
2.1 Understanding Human Behavior through Ergonomics
Ergonomics serves as a bridge between human behavior and system design. By observing and analyzing behavior in real-world or simulated environments, researchers can understand how people perform tasks, make decisions, and interact with their surroundings. This insight is crucial for improving user experiences, minimizing errors, and enhancing performance across industries.
2.1.1 Behavioral Insights in Ergonomics Research
In ergonomic research, understanding behavior involves more than just studying isolated actions. It requires analyzing the context in which behaviors occur, the goals of the user, the complexity of the task, and how external factors—like environmental conditions or task demands—impact performance.
- Behavioral Context: Human behavior in any system or environment is influenced by several factors, including the physical setup, the tools at hand, cognitive demands, and the user’s emotional state. Ergonomics aims to optimize these factors, enabling people to perform tasks more efficiently and safely.
- Example: In a manufacturing environment, the layout of a workstation can influence how quickly and accurately an operator performs a task. Adjusting the height of the workstation or rearranging tools to be within easy reach can improve productivity and reduce the risk of injury.
- Task Complexity and Cognitive Load: Cognitive load refers to the mental effort required to complete a task. High task complexity can overload the cognitive system, resulting in slower performance, increased stress, and higher error rates. Ergonomics research helps break down tasks into manageable components to minimize cognitive overload.
- Example: In healthcare settings, overly complex electronic health records (EHR) systems can overwhelm physicians, leading to missed diagnoses or incorrect entries. Simplifying the user interface through ergonomic design can improve accuracy and reduce the time spent on administrative tasks.
2.1.2 Methods for Assessing Behavior in Ergonomics
To effectively study human behavior within an ergonomic context, researchers employ a range of methods. These approaches can be observational, subjective (self-reporting), or objective (using biometric data and task performance metrics). Each method provides valuable insights into how people interact with systems and products, allowing researchers to make data-driven design decisions.
- Observational Studies: In observational research, human behavior is recorded and analyzed as users perform tasks in real-world settings or simulations. This method helps identify inefficiencies, patterns of error, or instances where the system is misaligned with human needs.
- Example: In retail, observing how customers navigate store layouts can help identify bottlenecks or poorly placed products. These insights can be used to optimize store design and improve the customer experience.
- Self-Reports and Surveys: Self-reports, through interviews or questionnaires, provide subjective data about users’ experiences, comfort levels, and perceived workload. While subjective, this data is crucial for understanding emotional or psychological responses that are not easily observable.
- Example: Pilots may report high stress levels when using overly complicated cockpit controls, despite performing their tasks successfully. This feedback can drive ergonomic improvements that reduce mental load.
- Objective Metrics: Biosensors and performance data offer objective measures of behavior, such as reaction times, error rates, and physiological responses (e.g., heart rate, eye movements). These metrics allow researchers to quantify the impact of ergonomic interventions.
- Example: Eye-tracking technology can objectively measure how users interact with website designs, revealing which elements capture their attention or cause confusion. This data helps improve web usability by focusing on intuitive navigation.
2.2 Human Error and Safety
One of the most important applications of Human Factors Ergonomics is understanding and mitigating human error. Human error is a significant contributor to accidents and inefficiencies across industries, from healthcare to aviation. By analyzing why errors occur, HFE can offer design solutions that minimize the likelihood of mistakes and enhance safety.
2.2.1 Types of Human Error
Human error can take several forms, each requiring different ergonomic solutions. Broadly, errors are classified as slips, lapses, mistakes, or violations. Understanding these categories is crucial for designing interventions that address the root causes of error.
- Slips and Lapses: Slips are unintended actions, such as pressing the wrong button, while lapses involve forgetting to complete a necessary step. Both types of errors typically occur due to distractions, fatigue, or confusing system designs.
- Example: In a control room setting, small, similar-looking buttons can lead to accidental presses (slips). Redesigning controls to differentiate critical functions can help prevent these errors.
- Mistakes: Mistakes occur when users make incorrect decisions based on poor information or flawed reasoning. Mistakes are often the result of complex, unclear, or unfamiliar system interfaces.
- Example: In medication administration, confusing labeling or similar packaging can lead to administering the wrong drug. Simplifying packaging designs can reduce the chance of such mistakes.
- Violations: Violations involve deliberate deviations from established procedures, often due to perceived inefficiencies or overconfidence. Ergonomics can address violations by redesigning tasks or systems to be more intuitive, so users are less likely to bypass them.
- Example: A worker might bypass a safety protocol if the process is seen as too time-consuming. Streamlining the protocol can improve compliance and reduce the risk of accidents.
2.2.2 Preventing Human Error through Ergonomic Design
Ergonomic interventions play a critical role in preventing human error by designing systems that are easier to understand, operate, and maintain. These interventions range from redesigning user interfaces to providing better feedback mechanisms that alert users when errors occur.
- Fail-Safes and Error Prevention: Ergonomic designs often include fail-safe mechanisms that prevent users from making catastrophic mistakes. These could be physical barriers, warning systems, or built-in redundancies.
- Example: In aviation, a fail-safe system prevents pilots from lowering landing gear at high speeds. This system reduces the risk of damage or accidents due to human error.
- Simplifying Interfaces: Overly complex systems increase the likelihood of mistakes. Streamlining interfaces and reducing the number of steps required to complete tasks can significantly reduce error rates.
- Example: In surgical settings, simplifying the layout of critical surgical tools can reduce the time it takes for the surgical team to respond to emergencies, improving patient outcomes.
2.3 Performance Optimization through Ergonomics
Human Factors Ergonomics is also focused on enhancing human performance by designing environments and systems that align with human strengths while compensating for limitations. Performance optimization is critical in high-demand industries, where efficiency, accuracy, and speed are essential.
2.3.1 Enhancing Performance in Complex Systems
Complex systems, such as those found in healthcare, aviation, and manufacturing, place significant cognitive and physical demands on users. Ergonomic interventions can help reduce these demands, improving overall performance.
- Workload Assessment and Balancing: Workload refers to the mental and physical effort required to perform a task. Overloading workers leads to fatigue and reduced performance, while underloading can result in disengagement and errors due to inattention. Ergonomic design aims to balance workload to keep individuals within an optimal performance zone.
- Example: In call centers, automated systems that handle repetitive inquiries can reduce cognitive load on employees, allowing them to focus on more complex tasks that require human judgment.
- Cognitive Aids and Automation: Automation and cognitive aids are used in ergonomic designs to support human performance. These aids can help manage the flow of information, reduce the need for multitasking, and minimize mental effort.
- Example: In emergency response centers, software that provides real-time data analysis and alerts helps dispatchers make informed decisions quickly, reducing response times and improving outcomes.
2.3.2 Managing Fatigue and Stress
Fatigue and stress are significant factors that degrade human performance. Long working hours, insufficient breaks, or overly demanding tasks can lead to exhaustion, increasing the likelihood of mistakes and reducing productivity. Ergonomics research provides solutions to monitor and manage fatigue and stress in real time.
- Fatigue Detection: Using biometric sensors like heart rate monitors, EEG, and eye-tracking devices, ergonomic systems can detect early signs of fatigue. These systems can then prompt breaks, adjust task demands, or alert supervisors to intervene.
- Example: In transportation, fatigue detection systems monitor driver alertness and issue warnings or adjust vehicle settings to prevent accidents caused by drowsy driving.
- Stress Management: Ergonomics also involves designing systems that minimize stress by reducing unnecessary complexity, providing clear feedback, and allowing for appropriate task pacing.
- Example: In high-pressure environments like trading floors, ergonomic designs that provide clear, real-time data in manageable formats help reduce stress and improve decision-making.
2.4 Tools and Techniques for Performance and Behavior Research
Understanding human behavior and optimizing performance require robust tools and techniques for data collection and analysis. Biosensors, behavioral observation tools, and simulation environments are commonly used in human factors research to gather objective data on user performance and system interactions.
2.4.1 Behavioral Observation Tools
- Video Recording and Analysis: Video footage is often used to capture user behavior in controlled environments. Researchers can analyze these recordings to assess performance, error rates, or how effectively users interact with a system.
- Example: In automotive research, video analysis of drivers can reveal how they respond to distractions or make split-second decisions in emergency scenarios.
2.4.2 Biometric Data Collection
- Eye Tracking: Eye-tracking technology allows researchers to analyze where users direct their visual attention, providing insight into cognitive workload, stress levels, and usability.
- Example: In website design, eye-tracking data shows which elements of a page draw the most attention, guiding designers in optimizing the layout for better user experience.
- Electroencephalography (EEG): EEG measures brain activity and is used to assess cognitive load, attention, and emotional states. This data is critical for understanding how users process information and react to stimuli in high-stakes environments.
- Example: In air traffic control, EEG is used to monitor mental workload during complex task management, allowing for real-time adjustments to prevent overload.
Chapter 3: Biosensors in Human Factors Research
The field of Human Factors Ergonomics (HFE) has evolved significantly with the advancement of technology, particularly through the integration of biosensors. These devices allow researchers to measure physiological and psychological responses in real-time, offering deeper insights into how individuals interact with systems, environments, and tasks. Biosensors provide objective data that can help researchers understand human behavior more comprehensively, enabling the development of ergonomic solutions that enhance performance, reduce stress, and prevent errors.
In this chapter, we will explore the range of biosensors used in human factors research, focusing on how these devices are employed to capture data related to cognitive load, stress, emotional responses, and physical strain. We will also discuss how biosensors contribute to optimizing design and improving safety across industries.
3.1 Introduction to Biosensors in Ergonomics
Biosensors are devices that detect and measure physiological changes in the body, providing real-time data about an individual’s emotional, cognitive, and physical state. In the context of human factors research, biosensors help quantify human interactions with systems, allowing for a more precise analysis of behavior, workload, stress, and fatigue. By using biosensors, researchers can assess how ergonomic interventions affect human performance and well-being, leading to more effective designs.
3.1.1 The Role of Biosensors in Ergonomic Research
Biosensors complement traditional human factors research methods, such as observational studies and self-reports, by offering objective measurements of physiological responses. These devices enable a more granular analysis of how individuals react to various conditions, such as high cognitive load, stress, or physical strain. Biosensors provide valuable data that helps researchers identify moments of peak stress, fatigue, or distraction, which are critical in designing systems that align with human capabilities and limitations.
- Example: In a usability study for a new software interface, biosensors can measure heart rate variability (HRV) and skin conductance (EDA) to detect stress levels when users encounter confusing elements, allowing designers to refine the interface for smoother interactions.
3.2 Key Biosensors in Human Factors Ergonomics
The integration of biosensors in human factors research allows for the collection of various physiological signals, each offering different insights into the user’s experience. In this section, we will discuss the most commonly used biosensors in ergonomics research and their applications.
3.2.1 Eye Tracking
Overview:
Eye-tracking technology records eye movements, including where and how long individuals focus their attention. This data is invaluable for understanding visual attention, cognitive load, and decision-making processes. By analyzing eye movements, researchers can determine how users interact with interfaces, products, or environments.
- Applications in Ergonomics:
- Visual Workload: Eye tracking helps assess the cognitive load during tasks requiring visual attention. By measuring the frequency and duration of fixations, researchers can determine if a task is too complex or if the layout of information is overwhelming.
- Interface Design: In software or website usability studies, eye tracking reveals how users navigate through visual content, helping to optimize layout, button placement, and design flow.
- Example: In an automotive environment, eye tracking is used to study driver distraction. Researchers can assess how often drivers shift their gaze from the road to in-vehicle displays, allowing for adjustments in dashboard design to minimize distractions.
3.2.2 Facial Expression Analysis
Overview:
Facial expression analysis uses computer vision and machine learning to detect and interpret facial movements that correspond to emotional states, such as happiness, surprise, frustration, or stress. This technology is particularly useful in understanding users’ emotional responses during interactions with products, systems, or environments.
- Applications in Ergonomics:
- Emotional Response Assessment: By analyzing micro-expressions, researchers can detect subtle emotional responses that indicate user satisfaction, engagement, or frustration with a system or task.
- Fatigue and Stress Monitoring: Facial expression analysis can detect signs of fatigue or stress, such as furrowed brows or a lack of emotional expressiveness, which can signal the need for intervention in high-demand work environments.
- Example: In customer experience research, facial expression analysis can track users’ emotional responses while interacting with a product, providing real-time feedback to improve design and usability.
3.2.3 Electrodermal Activity (EDA) / Galvanic Skin Response (GSR)
Overview:
EDA, also known as Galvanic Skin Response (GSR), measures changes in skin conductance that occur with emotional arousal. The higher the level of stress or excitement, the more the skin conducts electricity due to increased sweating. This biosensor is widely used in ergonomics research to assess emotional and physiological responses to various stimuli.
- Applications in Ergonomics:
- Stress and Arousal Monitoring: EDA is a reliable indicator of emotional arousal, allowing researchers to assess how different environments or tasks influence stress levels.
- Usability Testing: EDA is commonly used to evaluate user reactions to new systems or interfaces. A spike in skin conductance can indicate moments of confusion, frustration, or cognitive overload.
- Example: In gaming research, EDA sensors can detect how players react to certain in-game events or challenges, providing developers with insights into which game mechanics are exciting or stressful.
3.2.4 Electromyography (EMG)
Overview:
EMG measures electrical activity produced by skeletal muscles, allowing researchers to assess muscle strain, effort, and physical fatigue. This biosensor is particularly useful in understanding physical ergonomics and optimizing workstations, tools, and equipment to reduce musculoskeletal strain.
- Applications in Ergonomics:
- Posture and Fatigue Assessment: EMG is used to monitor muscle activity during physical tasks, helping identify postures or movements that cause excessive strain or fatigue. This information can be used to redesign workstations, tools, or tasks to prevent repetitive strain injuries.
- Tool Design: EMG data can guide the ergonomic design of tools and equipment to minimize muscle effort and reduce the risk of injury.
- Example: In assembly line work, EMG sensors can monitor muscle strain in the shoulders and back. If the data shows excessive strain, ergonomic adjustments can be made to the workstation or workflow to reduce the risk of musculoskeletal disorders.
3.2.5 Electroencephalography (EEG)
Overview:
EEG measures electrical activity in the brain, providing insights into cognitive states such as attention, mental workload, and fatigue. By analyzing brainwave patterns, researchers can understand how different tasks or environments affect cognitive performance.
- Applications in Ergonomics:
- Cognitive Workload Measurement: EEG is used to assess how demanding a task is on the brain. If brainwave patterns show signs of overload, tasks can be redesigned to reduce cognitive strain.
- Attention Monitoring: EEG can help track whether users are fully engaged with a task or if their attention is wandering, which is critical in high-stakes environments such as air traffic control or surgery.
- Example: In air traffic control, EEG can monitor controllers’ brain activity during high-traffic periods. If signs of mental fatigue are detected, the system can alert supervisors to allow for breaks, improving safety and performance.
3.2.6 Heart Rate (HR) and Heart Rate Variability (HRV)
Overview:
Heart rate (HR) and heart rate variability (HRV) are commonly used to assess stress, workload, and overall cardiovascular health. HR measures the number of heartbeats per minute, while HRV examines the variation in time between heartbeats, with higher variability generally indicating lower stress levels.
- Applications in Ergonomics:
- Stress and Workload Assessment: HR and HRV provide a reliable measure of physiological stress. An elevated heart rate or reduced HRV can indicate that a task is mentally or physically taxing, prompting ergonomic adjustments to reduce the burden.
- Fatigue Monitoring: A drop in HRV is often associated with fatigue. By monitoring HRV, researchers can assess when individuals are becoming fatigued, enabling interventions to improve safety and performance.
- Example: In military operations, HR and HRV are used to monitor soldiers’ stress levels during training exercises. If stress becomes too high, training can be adjusted to prevent cognitive overload and ensure optimal performance.
3.3 The Role of iMotions A/S in Multimodal Biosensor Integration
iMotions A/S is a leading provider of software that integrates data from multiple biosensors, offering researchers a comprehensive platform for analyzing human behavior in real-time. By synchronizing inputs from various sensors, iMotions enables a holistic understanding of how users interact with systems, making it an essential tool in human factors research.
3.3.1 Multimodal Data Collection and Analysis
The strength of iMotions lies in its ability to combine data from various biosensors—such as eye tracking, facial expression analysis, EEG, EDA, and EMG—into a single platform. This multimodal approach allows researchers to analyze physiological responses alongside behavioral data, providing a deeper understanding of user experiences.
- Example: In a usability study of a new medical device, iMotions can combine eye-tracking data (to see where users focus their attention), EDA data (to assess stress levels), and facial expression analysis (to gauge emotional responses). This holistic approach allows for a more comprehensive analysis of how users interact with the device, leading to better design improvements.
3.3.2 Real-Time Data Synchronization
One of the key features of the iMotions platform is real-time data synchronization, which allows researchers to capture and analyze multiple physiological signals as they occur. This capability is crucial in high-stakes environments where immediate feedback is necessary.
- Example: In a driving simulation, iMotions can collect data from eye trackers, HR sensors, and EEG devices simultaneously, allowing researchers to assess how drivers react to sudden changes in road conditions or distractions in real-time.
3.3.3 Data Visualization and Reporting
iMotions provides powerful data visualization tools that make it easier for researchers to interpret complex datasets. Heatmaps, time-synced graphs, and visual overlays help researchers identify patterns and draw meaningful conclusions from multimodal data.
- Example: In retail studies, iMotions can generate heatmaps based on eye-tracking data to show which parts of a store layout attract the most attention. Coupled with EDA data that shows emotional arousal, retailers can optimize store design to enhance customer experience.
Chapter 4: Software Solutions for Human Factors Ergonomics
The field of Human Factors Ergonomics (HFE) benefits immensely from technological advancements, particularly in the development of sophisticated software solutions that aid in data collection, analysis, and visualization. These tools enable researchers and ergonomics professionals to gather rich, multimodal data from various biosensors, analyze human behavior, and optimize systems for enhanced performance, safety, and user satisfaction.
iMotions A/S stands out as a leading provider of software tailored to human behavior research. With its multimodal platform, iMotions integrates a range of biosensors and provides researchers with the ability to track, synchronize, and analyze physiological, emotional, and behavioral data. This chapter will explore the software capabilities offered by iMotions and their relevance to human factors research.
4.1 Introduction to iMotions A/S
iMotions A/S is a pioneer in providing a complete software solution for human behavior research. The platform’s core functionality is the ability to collect and synchronize data from multiple biosensors, offering researchers a holistic view of how people interact with systems, environments, and products. iMotions combines data from eye trackers, facial expression analysis, electrodermal activity (EDA), electroencephalography (EEG), and more, delivering a unified solution for understanding human responses in real time.
4.1.1 The Importance of Multimodal Data Integration
Human behavior is complex, often involving simultaneous physiological, emotional, and cognitive responses. iMotions’ multimodal data integration allows researchers to capture this complexity, providing a clearer understanding of how users experience tasks and interact with systems.
- Example: During a driving simulation, iMotions can collect data from eye-tracking devices (to monitor where the driver is looking), heart rate monitors (to assess stress levels), and EEG (to gauge mental workload). By synchronizing all these inputs, the software offers a complete picture of the driver’s behavior and cognitive state during different driving scenarios.
4.2 Key Software Features of iMotions
The strength of iMotions lies in its comprehensive features, which allow for advanced data collection, analysis, and visualization. Researchers in the field of human factors ergonomics rely on these features to obtain actionable insights that can inform design and system improvements.
4.2.1 Multimodal Data Integration
iMotions supports the simultaneous collection of data from a wide range of biosensors, including:
- Eye Tracking to monitor visual attention.
- Facial Expression Analysis to detect emotional responses.
- EDA/GSR Sensors to track emotional arousal through skin conductance.
- EEG to measure cognitive workload and brain activity.
- Heart Rate and Heart Rate Variability to assess stress and workload.
- Electromyography (EMG) to capture muscle activity and physical strain.
These data streams are synchronized in real time, ensuring that researchers can analyze how different physiological responses correspond to specific tasks or stimuli.
- Example: In an office ergonomics study, iMotions can collect eye-tracking data to monitor how long employees focus on their screens, while simultaneously using EMG sensors to detect muscle tension in the neck and shoulders. If the data shows that prolonged screen time leads to increased muscle strain, researchers can recommend ergonomic interventions, such as breaks or improved posture.
4.2.2 Real-Time Data Collection and Monitoring
One of the most critical aspects of iMotions is its ability to collect data in real time. This feature is especially valuable in environments where immediate feedback is necessary, such as driving simulators, flight simulators, or clinical settings. Real-time monitoring enables researchers to track physiological and behavioral changes as they occur, allowing for dynamic adjustments and interventions.
- Example: In healthcare ergonomics, iMotions can monitor a surgeon’s cognitive load and stress levels during an operation using EEG and heart rate sensors. If the data indicates that the surgeon is becoming mentally fatigued, it could prompt the surgical team to consider taking breaks or adjusting the procedure to reduce cognitive strain.
4.2.3 Data Visualization and Reporting
iMotions offers robust data visualization tools that allow researchers to interpret complex data more easily. The software provides various visualization options, such as heatmaps (for eye-tracking data), emotional response timelines (for facial expression analysis), and real-time physiological graphs. These tools help researchers identify patterns, trends, and correlations within the data, making it easier to draw actionable insights.
- Heatmaps: Used in eye-tracking studies, heatmaps display where users focus their attention on a screen or in a physical environment. Areas that attract more attention are highlighted in warmer colors, providing an intuitive understanding of visual behavior.
- Example: In product design research, heatmaps can show which elements of packaging capture the most attention, guiding design decisions to emphasize key features or branding.
- Emotional Response Graphs: iMotions visualizes facial expression data in the form of time-synced emotional response graphs, which illustrate how a user’s emotions evolve throughout a task or interaction.
- Example: In a usability study, emotional response graphs can show moments of frustration or confusion, allowing designers to identify which parts of a system need improvement.
4.2.4 Customizable Workflows and Experiment Design
iMotions offers researchers flexibility in designing their experiments. The platform allows for the customization of workflows to suit specific research needs, including the ability to integrate self-reported measures (surveys, questionnaires) alongside biometric data. This flexibility ensures that the software can be tailored to different industries and research contexts.
- Example: In a consumer behavior study, researchers can use iMotions to combine facial expression analysis and eye-tracking data with self-reported surveys to measure emotional responses to product advertisements. By integrating both objective and subjective data, the software enables a deeper understanding of how consumers perceive the product.
4.3 iMotions Software Modules
iMotions offers several specialized modules that cater to different aspects of human factors ergonomics research. Each module is designed to work seamlessly with a variety of biosensors, allowing for detailed and specific analysis based on the research focus.
4.3.1 Eye Tracking Module
The eye-tracking module in iMotions integrates data from both screen-based and mobile eye-tracking systems, enabling researchers to study visual attention in a wide range of settings. The software tracks gaze points, fixations, and saccades, providing insights into how users interact with visual information.
- Applications:
- Web Usability: Understanding how users navigate websites and where they focus their attention.
- Product Design: Analyzing which elements of a product’s design capture attention or cause confusion.
- Example: In an automotive study, the eye-tracking module can be used to analyze where drivers direct their gaze while driving, helping to optimize the design of in-vehicle information systems and dashboard layouts.
4.3.2 Facial Expression Analysis Module
This module uses advanced algorithms to detect and classify facial expressions that correspond to basic emotions, such as happiness, surprise, anger, and fear. The module can track subtle changes in facial muscle movements, providing valuable data on how users emotionally respond to tasks or stimuli.
- Applications:
- User Experience (UX): Detecting frustration or satisfaction in response to software or product interactions.
- Customer Feedback: Measuring emotional reactions to advertising or marketing materials.
- Example: In a retail environment, facial expression analysis can reveal how customers emotionally react to product displays or advertisements, helping retailers optimize layouts and promotions to enhance engagement.
4.3.3 EEG Module
The EEG module in iMotions integrates with various EEG devices to measure brain activity. This module helps researchers assess cognitive states, such as attention, mental workload, and fatigue, offering insights into how different tasks impact cognitive performance.
- Applications:
- Cognitive Ergonomics: Understanding how users mentally process information in complex environments.
- Safety-Critical Environments: Monitoring cognitive workload in high-stress environments, such as aviation or surgery.
- Example: In a cognitive ergonomics study, EEG data can reveal whether a new user interface design reduces mental workload by showing changes in brainwave activity when users complete tasks.
4.3.4 EDA/GSR Module
The Electrodermal Activity (EDA) or Galvanic Skin Response (GSR) module measures changes in skin conductance, which indicate emotional arousal and stress. This data is invaluable in understanding how users react emotionally to different stimuli or environments.
- Applications:
- Usability Testing: Identifying moments of stress or confusion during interactions with software or devices.
- Marketing Research: Measuring emotional responses to advertisements or product features.
- Example: In a virtual reality (VR) study, EDA data can be used to measure how immersive or stressful a VR environment is, providing feedback to developers on how to improve user comfort and engagement.
4.3.5 Survey Module
The iMotions Survey Module allows researchers to combine objective biometric data with subjective self-reports, offering a more comprehensive view of user experience. Surveys can be administered during or after tasks, capturing user feedback on ease of use, comfort, or emotional response.
- Applications:
- Post-Task Feedback: Collecting user opinions on a system or product after they have interacted with it.
- Combining Data Streams: Integrating survey data with biometric measurements for a holistic understanding of user behavior.
- Example: In a workplace ergonomics study, researchers can use the Survey Module to gather feedback on perceived comfort while simultaneously measuring physiological data, such as muscle strain or heart rate variability.
4.4 Applications of iMotions Software Across Industries
The flexibility and comprehensive capabilities of iMotions make it applicable across numerous industries, where understanding human behavior is critical to improving design, safety, and performance. The following are some key industries where iMotions is used in human factors ergonomics research.
4.4.1 Healthcare
iMotions is used to monitor the cognitive load and stress levels of healthcare professionals, particularly in high-stakes environments such as surgery or emergency care. By integrating EEG, heart rate, and EDA data, researchers can identify moments of overload and suggest interventions to reduce fatigue and improve patient outcomes.
- Example: In operating rooms, iMotions can measure surgeons’ stress levels during complex procedures. The data helps optimize workflow and instrument layouts, improving efficiency and reducing cognitive strain.
4.4.2 Transportation
In the transportation industry, iMotions is used to study driver behavior, attention, and fatigue. By analyzing eye movements, EEG, and heart rate data, researchers can design vehicle systems that enhance safety and reduce the risk of accidents.
- Example: Automotive manufacturers use iMotions to assess how drivers interact with in-vehicle displays, adjusting the design to minimize distractions and maintain focus on the road.
4.4.3 Workplace Ergonomics
iMotions helps companies design better workspaces by collecting data on physical strain, posture, and cognitive load. By integrating EMG, EEG, and EDA data, researchers can identify which tasks or environments cause the most stress or fatigue, enabling ergonomic interventions to enhance productivity and well-being.
- Example: In office settings, iMotions can track employees’ posture and physical strain during long hours of computer use. Based on the data, ergonomic recommendations such as adjustable chairs or standing desks can be implemented to reduce discomfort and improve health.
Chapter 5: Applications of Human Factors Ergonomics in Different Industries
Human Factors Ergonomics (HFE) is a multidisciplinary field that spans across various industries, each requiring tailored solutions to optimize human-system interactions. The integration of ergonomics in these industries not only enhances user experience but also improves safety, performance, productivity, and well-being. From healthcare to consumer products, the principles of ergonomics guide the design of systems, environments, and tools to align with human capabilities and limitations. In this chapter, we will explore the diverse applications of HFE across key industries, highlighting how biosensors and software solutions, like iMotions, enable the refinement of ergonomically designed systems.
5.1 Healthcare
Healthcare environments are highly complex and demand extreme precision, quick decision-making, and continuous focus. In such settings, even minor ergonomic inefficiencies can lead to significant errors, jeopardizing patient safety and clinician well-being. The implementation of Human Factors Ergonomics in healthcare focuses on reducing cognitive and physical workload, improving medical device design, and enhancing overall system safety.
5.1.1 Ergonomics in Medical Device Design
Medical devices need to be designed with the end user in mind—whether it’s a healthcare professional or a patient. Devices that are difficult to use, require excessive force, or have complex interfaces can increase the risk of errors and injuries. Applying ergonomics in the design of medical devices helps reduce physical strain, improve usability, and ensure that the devices operate intuitively in high-stress situations.
- Example: When designing surgical tools, ergonomic considerations focus on grip comfort, weight distribution, and ease of movement. Tools that reduce muscle strain or prevent awkward postures during surgeries can enhance surgeon performance and reduce fatigue, leading to improved patient outcomes.
5.1.2 Monitoring Clinician Workload and Fatigue
Clinician fatigue and cognitive overload are major risk factors in healthcare settings, often contributing to medical errors. Biosensors, such as EEG and heart rate variability (HRV), are increasingly used to monitor the mental and physical workload of healthcare providers during critical tasks. These sensors provide real-time feedback that can help detect when a clinician is becoming fatigued or overwhelmed.
- Example: In an emergency room setting, iMotions can collect EEG and HRV data from doctors during their shifts to assess when they are reaching critical levels of fatigue. Based on this data, hospital administrators can adjust scheduling or implement protocols that allow for cognitive recovery, reducing the likelihood of errors caused by exhaustion.
5.1.3 Optimizing Operating Room Ergonomics
The design of operating rooms must be optimized for efficiency and safety. Poorly arranged tools, cluttered layouts, or improperly designed equipment can create distractions or require unnecessary movements, leading to delays or mistakes during surgery. Ergonomics helps streamline the design of surgical environments to minimize disruptions and improve focus.
- Example: Through eye tracking and EDA measurements, researchers can analyze how surgeons interact with tools, monitors, and staff during operations. Insights gained from these studies can lead to changes in the layout of operating rooms, ensuring that everything is within easy reach and visually accessible, ultimately enhancing surgical precision and patient safety.
5.2 Transportation
Transportation systems, from aviation to automotive, are highly reliant on human interaction. Whether it’s a pilot operating an aircraft or a driver navigating busy roads, ergonomic designs play a vital role in ensuring safety and efficiency. Human Factors Ergonomics focuses on optimizing vehicle controls, displays, and cockpit layouts to minimize cognitive and physical load while maximizing attention and reaction times.
5.2.1 Automotive Ergonomics: Enhancing Driver Safety and Comfort
In the automotive industry, ergonomics is applied to improve driver comfort and reduce distractions. Biosensors such as eye tracking and EEG are commonly used to study driver attention and alertness, while HRV sensors can detect signs of stress or fatigue.
- Example: iMotions can be used to track where drivers are looking during different driving conditions to ensure that dashboard layouts and heads-up displays provide necessary information without diverting attention from the road. By analyzing eye-tracking data, manufacturers can adjust the placement of controls and displays to minimize driver distraction.
5.2.2 Aviation: Cockpit Design and Cognitive Load Management
In aviation, cognitive workload and situational awareness are crucial factors affecting pilot performance. Cockpit designs must ensure that controls are intuitive, information is easily accessible, and pilots are not overwhelmed by excessive stimuli. Ergonomic principles are applied to reduce cognitive load, improve decision-making, and enhance safety.
- Example: iMotions software, combined with EEG and eye-tracking data, can be used to study how pilots manage complex flight scenarios, such as landing in adverse weather conditions. The data collected can inform redesigns of cockpit controls and information displays to improve pilot focus and reduce the chance of errors under stress.
5.2.3 Public Transportation: Ergonomic Design for Operators and Passengers
Public transportation systems, including buses, trains, and subways, rely on operators who face long hours of repetitive tasks, such as driving or monitoring systems. Ergonomics is applied to design operator workstations that reduce physical strain and fatigue, while also improving passenger experience through better seating, lighting, and accessibility.
- Example: EMG sensors can be used to monitor muscle strain in bus drivers, helping ergonomists design more comfortable seating and control layouts that reduce the risk of repetitive strain injuries. For passengers, studies using eye tracking and facial expression analysis can help optimize the design of seats and interior layouts to improve comfort and accessibility.
5.3 Workplace Ergonomics
The modern workplace, whether in offices or industrial environments, is constantly evolving. Ergonomics in the workplace aims to improve productivity, health, and job satisfaction by designing environments that reduce physical and mental strain, prevent injuries, and enhance employee well-being. Biosensors are increasingly used to monitor posture, stress, and fatigue, enabling real-time adjustments to workspace design.
5.3.1 Office Ergonomics: Reducing Strain and Improving Comfort
In office environments, ergonomics focuses on the design of workstations, including desk height, chair comfort, and the placement of monitors and keyboards. Poor ergonomic design can lead to musculoskeletal issues, eye strain, and mental fatigue, all of which reduce productivity and increase absenteeism.
- Example: iMotions can integrate eye-tracking and EMG sensors to study how employees interact with their workstations. If the data shows prolonged eye strain from poorly positioned monitors or muscle tension from improper desk height, ergonomic interventions such as adjustable desks, better lighting, or ergonomic chairs can be implemented to improve comfort and productivity.
5.3.2 Industrial Ergonomics: Preventing Injuries and Enhancing Productivity
In industrial settings, ergonomics is critical for preventing injuries caused by repetitive motions, heavy lifting, or awkward postures. Biosensors, such as EMG and motion capture systems, are used to analyze workers’ movements and physical exertion, helping design tools and workflows that reduce the risk of injury.
- Example: In an assembly line environment, EMG sensors can monitor muscle strain as workers perform repetitive tasks. If the data reveals excessive strain in certain muscle groups, adjustments can be made to the tool design or task flow, reducing the risk of long-term injuries like carpal tunnel syndrome or lower back pain.
5.3.3 Monitoring Employee Stress and Well-Being
Chronic stress and fatigue are common in many workplaces, leading to burnout and reduced performance. Biosensors, such as HRV and EEG, can monitor stress levels and cognitive load in real-time, helping employers detect when employees are becoming overwhelmed. Ergonomic interventions, such as adjustable work hours, stress-reducing environments, and better task management, can then be implemented.
- Example: In a high-demand work environment like customer support, iMotions can integrate EEG and heart rate data to monitor employee stress throughout the day. The data can inform shifts in workload, break scheduling, or the introduction of stress-relief practices, improving employee satisfaction and performance.
5.4 Consumer Products and Marketing
The design of consumer products has a direct impact on user experience, satisfaction, and purchasing decisions. Human Factors Ergonomics is critical in developing products that are intuitive, comfortable, and accessible for a broad range of users. Biosensors such as eye tracking, facial expression analysis, and EDA are used to assess how consumers interact with products, providing valuable feedback for designers and marketers.
5.4.1 Product Usability and Design
Ergonomically designed consumer products are easier to use, reduce physical strain, and improve user satisfaction. Whether it’s kitchen tools, electronics, or household appliances, ergonomics plays a key role in ensuring products are designed with human capabilities in mind.
- Example: iMotions can track how users handle a new kitchen appliance, measuring physical effort using EMG sensors and analyzing facial expressions to gauge satisfaction or frustration. Insights from these studies help designers refine product shapes, sizes, and control mechanisms to create more user-friendly designs.
5.4.2 Marketing Research: Understanding Consumer Behavior
Marketing researchers use ergonomics to understand how consumers emotionally engage with products, advertisements, or shopping environments. By analyzing emotional and physiological responses, marketers can optimize product displays, packaging, and branding to create stronger emotional connections with consumers.
- Example: Eye-tracking technology, combined with facial expression analysis through iMotions, can be used to study how consumers visually engage with product packaging in a retail setting. By identifying which design elements capture attention and which evoke positive emotions, brands can refine their packaging to increase appeal and sales.
5.5 Gaming and Entertainment
The gaming and entertainment industry leverages Human Factors Ergonomics to create immersive, intuitive, and engaging experiences for users. Whether designing gaming controllers, virtual reality (VR) systems, or interactive displays, ergonomics ensures that users can interact with technology in a way that enhances their enjoyment and comfort while minimizing physical and cognitive strain.
5.5.1 Ergonomic Design in Gaming Interfaces
Gaming controllers and interfaces must be designed for long-term use without causing physical discomfort. Ergonomics plays a critical role in designing controllers that fit comfortably in the hands, with buttons and joysticks placed optimally for ease of use.
- Example: Using EMG sensors to monitor muscle activity in the hands, iMotions can help game developers identify how different controller designs affect comfort and performance. This data can lead to improvements in controller ergonomics, ensuring that gamers can play for extended periods without experiencing fatigue or strain.
5.5.2 Virtual Reality (VR) and Immersive Experiences
In VR and augmented reality (AR), ergonomics is essential to creating immersive experiences without causing discomfort, disorientation, or motion sickness. Eye tracking, EDA, and EEG sensors are used to measure how users engage with virtual environments, providing feedback on how to improve comfort and reduce cognitive overload.
- Example: iMotions can track users’ eye movements and emotional responses while navigating a virtual world, allowing developers to adjust elements like visual clarity, movement controls, and scene transitions to ensure a more comfortable and engaging experience.
Chapter 6: Challenges and Future Directions in Human Factors Ergonomics
As technology advances and the complexity of human-system interactions increases, Human Factors Ergonomics (HFE) faces new challenges and opportunities. This chapter explores the key issues currently shaping the field, including ethical considerations, accessibility, and the balance between human and technological capabilities. Additionally, we will discuss emerging trends, such as the growing role of artificial intelligence (AI), machine learning, and neuroergonomics, that are likely to redefine how ergonomics is applied in research and design.
6.1 Challenges in Human Factors Ergonomics
Despite the significant advances in HFE, the field still faces numerous challenges. These range from the ethical use of biometric data to the difficulties of designing for increasingly diverse populations and ensuring that the systems we create are both safe and intuitive for all users.
6.1.1 Ethical Considerations in the Use of Biosensors
The integration of biosensors in human factors research has provided unprecedented insights into human behavior, but it has also raised important ethical questions. Collecting real-time physiological data, such as heart rate, brain activity, and emotional responses, involves sensitive information about individuals’ mental and physical states. The ethical management of this data is critical.
- Data Privacy and Security: One of the primary ethical concerns in HFE is ensuring that the data collected from biosensors is stored, processed, and shared securely. Participants need to be assured that their personal data will not be misused or exposed to unauthorized entities.
- Example: In a workplace setting where EEG is used to monitor cognitive load and fatigue, employees must be fully informed about how their brain activity data will be used, and safeguards must be put in place to prevent unauthorized access to this sensitive information.
- Informed Consent: Participants in studies using biosensors must provide informed consent, meaning they understand the type of data being collected, how it will be used, and the potential risks involved. Special care must be taken to ensure that vulnerable populations, such as children or individuals with disabilities, understand these risks.
- Balancing Research Insights and Privacy: While biosensors provide valuable insights into behavior, it is important to consider how much monitoring is necessary. Excessive or invasive data collection can lead to discomfort or distrust among participants. Researchers need to strike a balance between gathering enough data to gain useful insights and respecting individual privacy.
6.1.2 Accessibility and Inclusive Design
Designing systems and environments that are accessible to all users, regardless of their physical, sensory, or cognitive abilities, remains a significant challenge in HFE. Ergonomics must address the diverse needs of users by ensuring that systems are adaptable and inclusive, providing equal access and usability for all.
- Physical Disabilities: People with physical impairments may struggle to interact with systems that are not designed with their needs in mind. Adjustable workstations, voice-activated controls, and haptic feedback are examples of ergonomic solutions that can make systems more accessible.
- Example: A person with limited mobility might benefit from an adjustable desk that can be easily lowered or raised to accommodate both sitting and standing positions, reducing strain and improving accessibility.
- Cognitive and Sensory Impairments: Individuals with cognitive disabilities, such as reduced memory capacity or slower reaction times, as well as those with sensory impairments, such as vision or hearing loss, require systems that can be customized to meet their needs.
- Example: Websites and software applications can be made more accessible by offering text-to-speech functionality, scalable fonts, and simplified navigation for users with visual impairments.
6.1.3 Designing for Complex Systems
As human-machine systems become more complex, ergonomists face the challenge of designing intuitive interfaces that reduce cognitive overload. In industries like healthcare, aviation, and military operations, operators are required to process vast amounts of information in real-time, often under stressful conditions. Designing systems that simplify complex tasks while maintaining safety and efficiency is a growing challenge.
- Example: In an air traffic control environment, ergonomic designs need to reduce the cognitive load on controllers by presenting information in a clear and organized manner, ensuring that critical data is easy to access during high-pressure situations.
6.2 Future Trends in Human Factors Ergonomics
The future of Human Factors Ergonomics will be shaped by advances in technology, particularly AI, machine learning, neuroergonomics, and immersive technologies like virtual reality (VR) and augmented reality (AR). These innovations will offer new ways to understand and optimize human behavior, but they will also require new approaches to ergonomics.
6.2.1 Artificial Intelligence and Machine Learning in Ergonomics
AI and machine learning are transforming how human factors research is conducted and applied. These technologies can analyze large datasets to predict user behavior, identify patterns, and suggest design improvements. AI-driven ergonomic systems can adapt in real-time, personalizing user experiences based on physiological and behavioral data.
- AI in Predicting Human Behavior: Machine learning algorithms can analyze multimodal biometric data to predict user behavior, such as when a user is likely to become fatigued, distracted, or stressed. This allows systems to adjust dynamically to user states, improving safety and performance.
- Example: In a smart manufacturing environment, AI-driven systems can monitor workers’ physical and cognitive states using biosensors. When the system detects signs of fatigue or declining performance, it can adjust the workload, suggest breaks, or even reassign tasks to optimize productivity and safety.
- Automation of Ergonomic Assessments: AI can automate the assessment of ergonomic risks by analyzing video footage of workers or using sensors to detect unsafe postures and movements. This reduces the need for manual ergonomic audits and provides real-time insights for interventions.
- Example: In a warehouse setting, AI systems can automatically analyze workers’ lifting techniques, identifying when they are at risk of injury due to poor posture or excessive strain, and providing real-time feedback to correct these issues.
6.2.2 Neuroergonomics: The Intersection of Neuroscience and Ergonomics
Neuroergonomics is an emerging field that combines neuroscience with ergonomics to study brain function in relation to work tasks, system interactions, and environments. By using brain imaging techniques such as EEG and functional near-infrared spectroscopy (fNIRS), neuroergonomics seeks to design environments and systems that are in harmony with the brain’s natural processing abilities.
- Applications in Cognitive Workload Management: Neuroergonomics is particularly useful in managing cognitive workload. By understanding how the brain responds to different tasks, researchers can design systems that reduce mental overload, improve decision-making, and enhance overall cognitive performance.
- Example: In control rooms, EEG and fNIRS sensors can monitor operators’ brain activity to detect when they are becoming mentally fatigued. The system can then adjust task demands, reassign responsibilities, or introduce breaks to prevent errors caused by cognitive overload.
- Brain-Computer Interfaces (BCIs): BCIs allow users to control systems using brain signals, bypassing traditional input methods like keyboards or touchscreens. This technology has significant potential in assisting individuals with disabilities, enabling them to interact with systems more effectively and efficiently.
- Example: A person with limited mobility could use a BCI to control their environment, such as adjusting the lighting or operating household appliances, simply by thinking about the action, providing a new level of accessibility.
6.2.3 Immersive Technologies: Virtual Reality (VR) and Augmented Reality (AR)
VR and AR technologies are increasingly being used in ergonomics for training, simulations, and design testing. These immersive environments provide researchers with a controlled space to observe human behavior and test ergonomic designs before implementing them in real-world settings.
- Ergonomic Design Testing in Virtual Environments: VR allows researchers to test different workspace layouts, tool designs, and system interfaces in a simulated environment. This enables rapid iteration and refinement of designs before they are implemented in physical environments.
- Example: In an industrial design study, VR can simulate different workstation setups, allowing workers to test the ergonomics of each layout without needing physical prototypes. This accelerates the design process and ensures that the final product is optimized for human use.
- Training and Skill Development: AR can enhance training by overlaying real-time information or guidance on a worker’s view. This can help improve task performance, reduce errors, and shorten training times.
- Example: In maintenance operations, AR headsets can guide workers through complex tasks by overlaying step-by-step instructions on the equipment they are repairing, reducing cognitive load and improving efficiency.
6.3 The Role of iMotions in Shaping the Future of Human Factors Ergonomics
As the future of HFE unfolds, iMotions is positioned to play a key role in advancing the field through its powerful multimodal data collection and analysis platform. By integrating cutting-edge biosensor technology with AI-driven analysis, iMotions enables researchers and designers to gain deeper insights into human behavior and system interactions.
6.3.1 Expanding Multimodal Research Capabilities
As more biosensors and behavioral data streams become available, iMotions will continue to expand its capabilities to integrate and synchronize diverse data sources. This will provide researchers with an even richer understanding of how different physiological, cognitive, and emotional factors influence human behavior in complex environments.
- Example: In future work environments, iMotions may integrate new types of biosensors, such as wearables that monitor hydration levels or muscle fatigue, allowing for more comprehensive ergonomic assessments and personalized interventions to enhance worker well-being.
6.3.2 Leveraging AI for Predictive Ergonomics
iMotions can harness the power of AI to predict ergonomic risks and suggest interventions in real time. By analyzing historical and real-time data, AI algorithms can identify patterns that indicate when users are likely to experience fatigue, stress, or cognitive overload, enabling dynamic adjustments to task demands and environments.
- Example: In healthcare, iMotions could integrate predictive algorithms to monitor surgeons’ stress levels during an operation. If the system detects an increased risk of error due to cognitive fatigue, it could alert the surgical team or suggest task-sharing to maintain patient safety.
6.3.3 Supporting Ethical and Inclusive Research
As the ethical challenges around biometric data collection and privacy grow, iMotions will continue to prioritize data security and ethical considerations. By building robust consent protocols, anonymization techniques, and data protection measures into its platform, iMotions can help ensure that future ergonomic research is conducted responsibly.
Appendix
A. Key Ergonomic Standards and Guidelines
A variety of international standards and guidelines help ensure that ergonomic principles are applied consistently and effectively across different industries. Below is a summary of the most widely used standards.
- ISO 9241 – Ergonomics of Human-System Interaction: This standard covers various aspects of user-centered design for interactive systems, including usability, visual ergonomics, and input devices.
- ANSI/HFES 100 – Human Factors Engineering of Computer Workstations: This standard provides guidelines for the design of computer workstations to minimize discomfort and reduce the risk of musculoskeletal disorders.
- ISO 6385 – Ergonomic Principles in the Design of Work Systems: This standard outlines general ergonomic principles to optimize the design of work systems, focusing on human well-being and performance.
- OSHA Guidelines for Ergonomics: The U.S. Occupational Safety and Health Administration (OSHA) provides guidelines aimed at preventing workplace injuries, focusing on the reduction of repetitive strain injuries (RSIs) and musculoskeletal disorders.
- ISO 45001 – Occupational Health and Safety Management Systems: This international standard helps organizations reduce workplace injuries and provides a framework for improving worker safety, including ergonomic considerations.
- European Standard EN 1335 – Office Furniture – Office Work Chair Requirements: This standard defines ergonomic requirements for office chairs to ensure comfort and prevent back problems in office environments.
B. Glossary of Key Terms in Human Factors Ergonomics
- Anthropometry: The study of human body measurements, often used in ergonomic design to ensure that products fit the intended user population.
- Cognitive Load: The amount of mental effort required to perform a task. High cognitive load can lead to errors or reduced performance, while low cognitive load can increase efficiency.
- Electrodermal Activity (EDA): A measure of changes in the skin’s conductance caused by sweat, often used to assess emotional arousal and stress.
- Electromyography (EMG): A technique that measures electrical activity in muscles, used in ergonomics to analyze physical strain and fatigue.
- Electroencephalography (EEG): A method of measuring brain activity through electrical signals, commonly used to assess cognitive workload and attention.
- Eye Tracking: A technology that tracks where a person is looking, helping researchers understand visual attention and workload.
- Human-System Interaction (HSI): The study of how people interact with systems, focusing on optimizing usability, safety, and performance.
- Neuroergonomics: A field that integrates neuroscience with ergonomics, focusing on brain activity in relation to system design and performance.
- Repetitive Strain Injury (RSI): An injury caused by repetitive motions or awkward postures, often preventable through ergonomic interventions.
- Usability: The ease with which a user can interact with a system or product, often a key focus in ergonomic design.
- Workstation Design: The arrangement and setup of tools, equipment, and furniture to optimize comfort and productivity, minimizing the risk of strain or injury.
C. Resources for Further Reading
For readers who wish to dive deeper into the topics covered in this guide, the following books, journal articles, and reports provide comprehensive insights into Human Factors Ergonomics:
- Books:
- Human Factors in Engineering and Design by Mark S. Sanders and Ernest J. McCormick – A foundational text on the application of ergonomics principles in design.
- Designing for People by John D. Gould – This book explores the principles of user-centered design, emphasizing usability and system interaction.
- The Measure of Man and Woman: Human Factors in Design by Alvin R. Tilley – A key reference on anthropometric data and its application in ergonomic design.
- Journal Articles:
- Wickens, C.D., “Processing Resources in Attention,” Cognitive Psychology, 1984 – A classic paper that explores how humans process information and how cognitive load affects performance.
- Carayon, P., “Human Factors of Complex Work Systems in Healthcare,” Journal of Human Factors and Ergonomics in Healthcare, 2010 – This article discusses the challenges of applying ergonomic principles in high-complexity environments like healthcare.
- Straker, L., Mathiassen, S.E., “Increased Physical Workloads in Modern Office Work: A Challenge for Ergonomics,” Applied Ergonomics, 2009 – A review of ergonomic challenges in office work environments as technology evolves.
- Reports:
- World Health Organization (WHO) – Ergonomics and Workplace Health (2013): A global overview of the impact of ergonomics on worker health and productivity.
- Human Factors and Ergonomics Society Annual Report: A yearly publication that summarizes advancements and trends in HFE research and applications.
iMotions Ergonomics Research Lab
Synchronize data collection from multiple biosensors, allowing researchers to investigate complex research questions in innovative and time saving ways.
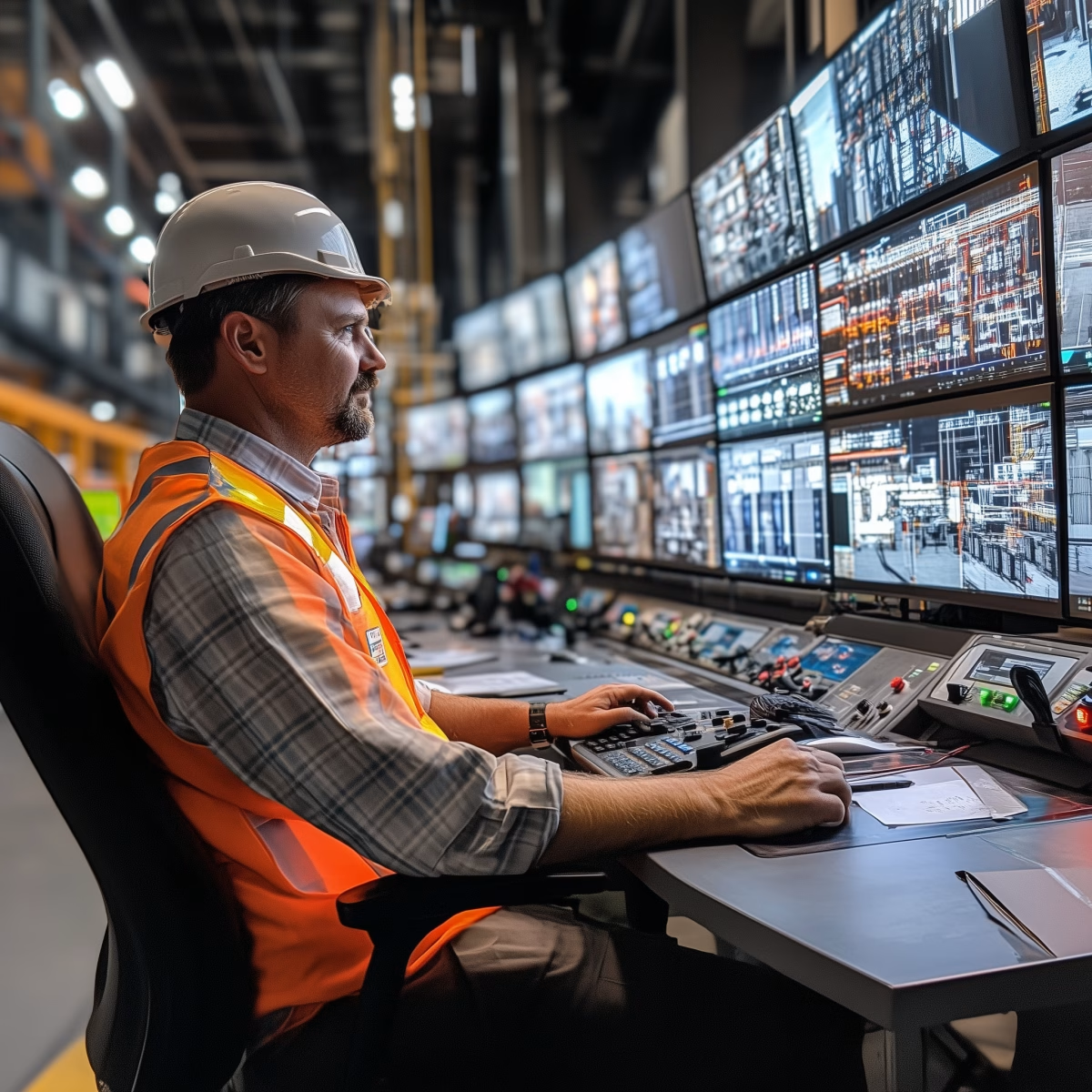